Electrical breakdown
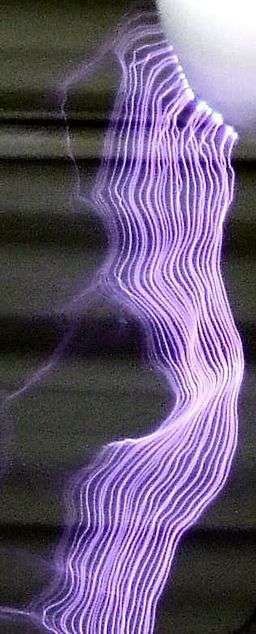
Electrical breakdown or dielectric breakdown is a long reduction in the resistance of an electrical insulator when the voltage applied across it exceeds the breakdown voltage. This results in the insulator becoming electrically conductive. Electrical breakdown may be a momentary event (as in an electrostatic discharge), or may lead to a discontinuous arc charge if protective devices fail to interrupt the current in a low power circuit.
Under sufficient electrical stress, electrical breakdown can occur within solids, liquids, gases or vacuum. However, the specific breakdown mechanisms are significantly different for each, particularly in different kinds of dielectric medium.
Failure of electrical insulation
Electrical breakdown is often associated with the failure of solid or liquid insulating materials used inside high voltage transformers or capacitors in the electricity distribution grid, usually resulting in a short circuit or a blown fuse. Electrical breakdown can also occur across the insulators that suspend overhead power lines, within underground power cables, or lines arcing to nearby branches of trees.
Mechanism
Breakdown mechanisms seem to differ in solids, liquids, and gasses. Breakdown is influenced by electrode material, sharp curvature of conductor material (resulting in locally intensified electric fields), the size of the gap between the electrodes, and the density of the material in the gap.
Breakdown in solids
In solid materials (e.g. power cables) a long-time partial discharge typically precedes breakdown, degrading the insulators and metals nearest the voltage gap. Ultimately the partial discharge chars through a channel of carbonized material that conducts current across the gap.
Breakdown in liquids
There are several possible mechanisms for breakdown in liquids: bubbles, small impurities, and electrical super-heating have all been proposed. The process of breakdown in liquids seems to be more complicated because of hydrodynamic effects, since additional pressure is exerted on the fluid by the non-linear electrical field strength in the gap between the electrodes.
- In liquefied gases used as coolants for superconductivity – such as Helium at 4.2 K or Nitrogen at 96 K – bubbles should induce breakdown.
- In oil-cooled and oil-insulated transformers the field strength for breakdown is about 20 kV/mm (as compared to 3 kV/mm for dry air). Despite the purified oils used, small particle contaminants are blamed.
Breakdown in gasses
Thermal effects are proposed for gasses by some authors, since breakdown in 50/60 Hz AC lines sometimes occurs long after the maximum voltage is reached.
Electrical breakdown occurs within a gas (or mixture of gases, such as air) when the dielectric strength of the gas is exceeded. Regions of intense voltage gradients can cause nearby gas to partially ionize and begin conducting. This is done deliberately in low pressure discharges such as in fluorescent lights (see also Electrostatic discharge) or in an electrostatic precipitator. The voltage that leads to electrical breakdown of a gas is approximated by Paschen's Law.
Partial electrical breakdown of the air causes the "fresh air" smell of ozone during thunderstorms or around high-voltage equipment. Although air is normally an excellent insulator, when stressed by a sufficiently high voltage (an electric field strength of about 3 x 106 V/m or 3 kV/mm[1]), air can begin to break down, becoming partially conductive. Across relatively small gaps, breakdown voltage in air is a function of gap length times pressure. If the voltage is sufficiently high, complete electrical breakdown of the air will culminate in an electrical spark or an electric arc that bridges the entire gap.
The color of the spark depends upon the gases that make up the gaseous media. While the small sparks generated by static electricity may barely be audible, larger sparks are often accompanied by a loud snap or bang. Lightning is an example of an immense spark that can be many miles long.
Persistent arcs
If a fuse or circuit breaker fails to interrupt the current through a spark in a power circuit, current may continue, forming a very hot electric arc (about 30 000 degrees). The color of an arc depends primarily upon the conducting gasses, some of which may have been solids before being vaporized and mixed into the hot plasma in the arc. The free ions in and around the arc recombine to create new chemical compounds, such as ozone, carbon monoxide, and nitrous oxide. Ozone is most easily noticed due to its distinct odour.[2]
Although sparks and arcs are usually undesirable, they can be useful in everyday applications such as spark plugs for gasoline engines, electrical welding of metals, or for metal melting in an electric arc furnace. Prior to gas discharge the gas glows with distinct colors that depend on the energy levels of the atoms. Recent US research using gamma ray observatories in the orbit have shown that breakdown is influenced by gamma rays reaching up to thousand kilometers. Not all mechanisms are fully understood.
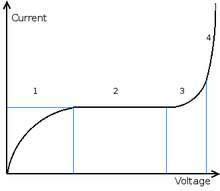
The vacuum itself is expected to undergo electrical breakdown at or near the Schwinger limit.
Voltage-current relation
Before gas breakdown, there is a non-linear relation between voltage and current as shown in the figure. In region 1, there are free ions that can be accelerated by the field and induce a current. These will be saturated after a certain voltage and give a constant current, region 2. Region 3 and 4 are caused by ion avalanche as explained by the Townsend discharge mechanism.
Friedrich Paschen established the relation between the breakdown condition to breakdown voltage. He derived a formula that defines the breakdown voltage () for uniform field gaps as a function of gap length () and gap pressure ().[3]
Paschen also derived a relation between the minimum value of pressure gap for which breakdown occurs with a minimum voltage.[3]
and are constants depending on the gas used.
Corona breakdown
Partial breakdown of the air occurs as a corona discharge on high voltage conductors at points with the highest electrical stress. As the dielectric strength of the material surrounding the conductor determines the maximum strength of the electric field the surrounding material can tolerate before becoming conductive, conductors that consist of sharp points, or balls with small radii, are more prone to causing dielectric breakdown, because the field strength of tips is much higher than that of a plate capacitor (homogeneous field).
Appearance
Corona is sometimes seen as a bluish glow around high voltage wires and heard as a sizzling sound along high voltage power lines. Corona also generates radio frequency noise that can also be heard as ‘static’ or buzzing on radio receivers. Corona can also occur naturally as “St. Elmo's Fire” at high points such as church spires, treetops, or ship masts during thunderstorms.
Ozone generation
Corona discharge ozone generators have been used for more than 30 years in the water purification process. Ozone is a toxic gas, even more potent than chlorine. In a typical drinking water treatment plant, the ozone gas is dissolved into the filtered water to kill bacteria and destroy viruses. Ozone also removes the bad odours and taste from the water. The main advantage of ozone is that any residual overdose decomposes to gaseous oxygen well before the water reaches the consumer. This is in contrast with chlorine gas or chlorine salts, which stay in the water longer and can be tasted by the consumer.
Other uses
Although corona discharge is usually undesirable, until recently it was essential in the operation of photocopiers (xerography) and laser printers. Many modern copiers and laser printers now charge the photoconductor drum with an electrically conductive roller, reducing undesirable indoor ozone pollution.
Lightning rods use corona discharge to create conductive paths in the air that point towards the rod, deflecting potentially-damaging lightning away from buildings and other structures.[4]
Corona discharges are also used to modify the surface properties of many polymers. An example is the corona treatment of plastic materials which allows paint or ink to adhere properly.
Disruptive devices
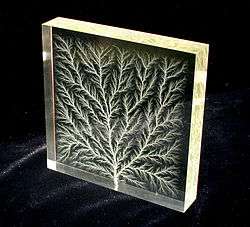
A disruptive device is designed to electrically overstress a dielectric beyond its dielectric strength so as to intentionally cause electrical breakdown of the device. The disruption causes a sudden transition of a portion of the dielectric, from an insulating state to a highly conductive state. This transition is characterized by the formation of an electric spark or plasma channel, possibly followed by an electric arc through part of the dielectric material.
If the dielectric happens to be a solid, permanent physical and chemical changes along the path of the discharge will significantly reduce the material's dielectric strength, and the device can only be used one time. However, if the dielectric material is a liquid or gas, the dielectric can fully recover its insulating properties once current through the plasma channel has been externally interrupted.
Commercial Spark gaps use this property to abruptly switch high voltages in pulsed power systems, to provide surge protection for telecommunication and electrical power systems, and ignite fuel via spark plugs in many internal combustion engines. Spark gaps also were used in early radio-telegraph transmitters to generate radio-frequency oscillations.
See also
- Discharges
- Electrostatic discharge, corona discharge, Partial discharge, Avalanche breakdown, Glow discharge, Direct current discharge, Lichtenberg figure, Electrical treeing, Townsend discharge, Arc Flash Paschen's law
References
- ↑ Hong, Alice (2000). "Dielectric Strength of Air". The Physics Factbook.
- ↑ "Lab Note #106 Environmental Impact of Arc Suppression". Arc Suppression Technologies. April 2011. Retrieved March 15, 2012.
- 1 2 Ray, Subir (2009). An Introduction to High Voltage Engineering. PHI Learning. pp. 19–21. ISBN 978-8120324176.
- ↑ Young, Hugh D.; Roger A. Freedman; A. Lewis Ford (2004) [1949]. "Electric Potential". Sears and Zemansky's University Physics (11 ed.). San Francisco: Addison Wesley. pp. 886–7. ISBN 0-8053-9179-7.