Sodium-cooled fast reactor
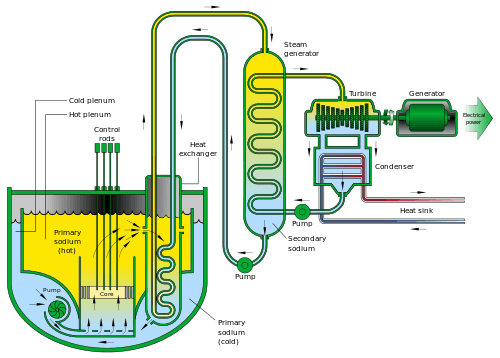
The sodium-cooled fast reactor (SFR) is a Generation IV reactor project to design an advanced fast neutron reactor.
It builds on two closely related existing projects, the LMFBR and the Integral Fast Reactor, with the objective of producing a fast-spectrum, sodium-cooled reactor.
The reactors are intended for use in nuclear power plants to produce nuclear power from nuclear fuel.
Fuel cycle
The nuclear fuel cycle employs a full actinide recycle with two major options: One is an intermediate-size (150–600 MWe) sodium-cooled reactor with uranium-plutonium-minor-actinide-zirconium metal alloy fuel, supported by a fuel cycle based on pyrometallurgical reprocessing in facilities integrated with the reactor. The second is a medium to large (500–1,500 MWe) sodium-cooled reactor with mixed uranium-plutonium oxide fuel, supported by a fuel cycle based upon advanced aqueous processing at a central location serving a number of reactors. The outlet temperature is approximately 510–550 degrees Celsius for both.
Sodium as a coolant
Liquid metallic sodium may be used as the sole coolant, carrying heat from the core. Sodium has only one stable isotope, sodium-23. Sodium-23 is a very weak absorber of neutrons. When it does absorb a neutron it produces sodium-24, which has a half-life of 15 hours and decays in to magnesium-24.
Advantages

An advantage of liquid metal coolants is that despite low specific heat, sodium melts at 371K and boils / vaporizes at 1156K, allowing a total "temperature outlier" range of 785K of heat variation between solid / frozen and gas / vapor states allowing the absorption of significant heat, less safety margins, in liquid phase. The high thermal conductivity properties effectively create a reservoir of heat capacity which provides thermal inertia against overheating.[1] Water is difficult to use as a coolant for a fast reactor because water acts as a neutron moderator that slows the fast neutrons into thermal neutrons. Unlike liquid sodium, water has a higher specific heat, with a smaller liquid range of just 100K between ice and gas at normal, sea-level atmospheric pressure conditions. While it may be possible to use supercritical water as a coolant in a fast reactor, this would require a very high pressure. In contrast, sodium atoms are much heavier than both the oxygen and hydrogen atoms found in water, and therefore the neutrons lose less energy in collisions with sodium atoms. Sodium also need not be pressurized since its boiling point is much higher than the reactor's operating temperature, and sodium does not corrode steel reactor parts.[1] The high temperatures reached by the coolant (up to 1156K for pure molten sodium, less all Generation IV margins of alarm call safety) permit a higher thermodynamic efficiency than in water cooled reactors.[2] The molten sodium, being electrically conductive, can be pumped by electromagnetic pumps.[2]
Disadvantages
A disadvantage of sodium is its chemical reactivity, which requires special precautions to prevent and suppress fires. If sodium comes into contact with water it explodes, and it burns when in contact with air. This was the case at the Monju Nuclear Power Plant in a 1995 accident. In addition, neutrons cause it to become radioactive; however, activated sodium has a half-life of only 15 hours.[1]
Design goals
The operating temperature should not exceed the melting temperature of the fuel. Fuel-to-cladding chemical interaction (FCCI) has to be designed against. FCCI is eutectic melting between the fuel and the cladding; uranium, plutonium, and lanthanum (a fission product) inter-diffuse with the iron of the cladding. The alloy that forms has a low eutectic melting temperature. FCCI causes the cladding to reduce in strength and could eventually rupture. The amount of transuranic transmutation is limited by the production of plutonium from uranium. A design work-around has been proposed to have an inert matrix. Magnesium oxide has been proposed as the inert matrix. Magnesium oxide has an entire order of magnitude smaller probability of interacting with neutrons (thermal and fast) than elements like iron.[3]
Actinides and fission products by half-life | ||||||||
---|---|---|---|---|---|---|---|---|
Actinides[4] by decay chain | Half-life range (y) |
Fission products of 235U by yield[5] | ||||||
4n | 4n+1 | 4n+2 | 4n+3 | |||||
4.5–7% | 0.04–1.25% | <0.001% | ||||||
228Ra№ | 4–6 | † | 155Euþ | |||||
244Cmƒ | 241Puƒ | 250Cf | 227Ac№ | 10–29 | 90Sr | 85Kr | 113mCdþ | |
232Uƒ | 238Puƒ№ | 243Cmƒ | 29–97 | 137Cs | 151Smþ | 121mSn | ||
248Bk[6] | 249Cfƒ | 242mAmƒ | 141–351 |
No fission products | ||||
241Amƒ | 251Cfƒ[7] | 430–900 | ||||||
226Ra№ | 247Bk | 1.3 k – 1.6 k | ||||||
240Puƒ№ | 229Th№ | 246Cmƒ | 243Amƒ | 4.7 k – 7.4 k | ||||
245Cmƒ | 250Cm | 8.3 k – 8.5 k | ||||||
239Puƒ№ | 24.1 k | |||||||
230Th№ | 231Pa№ | 32 k – 76 k | ||||||
236Npƒ | 233Uƒ№ | 234U№ | 150 k – 250 k | ‡ | 99Tc₡ | 126Sn | ||
248Cm | 242Puƒ | 327 k – 375 k | 79Se₡ | |||||
1.53 M | 93Zr | |||||||
237Npƒ№ | 2.1 M – 6.5 M | 135Cs₡ | 107Pd | |||||
236U№ | 247Cmƒ | 15 M – 24 M | 129I₡ | |||||
244Pu№ | 80 M |
... nor beyond 15.7 M years[8] | ||||||
232Th№ | 238U№ | 235Uƒ№ | 0.7 G – 14.1 G | |||||
Legend for superscript symbols |
The SFR is designed for management of high-level wastes and, in particular, management of plutonium and other actinides. Important safety features of the system include a long thermal response time, a large margin to coolant boiling, a primary system that operates near atmospheric pressure, and intermediate sodium system between the radioactive sodium in the primary system and the water and steam in the power plant. With innovations to reduce capital cost, such as making a modular design, removing a primary loop, integrating the pump and intermediate heat exchanger, or simply find better materials for construction, the SFR can be a viable technology for electricity generation.[9]
The SFR's fast spectrum also makes it possible to use available fissile and fertile materials (including depleted uranium) considerably more efficiently than thermal spectrum reactors with once-through fuel cycles.
Reactors
Sodium-cooled reactors have included:
|
|
Most of these were experimental plants, which are no longer operational
Related:
- Fast Flux Test Facility, United States, a sodium-cooled fast neutron reactor
See also
References
- 1 2 3 Fanning, Thomas H. (May 3, 2007). "Sodium as a Fast Reactor Coolant" (PDF). Topical Seminar Series on Sodium Fast Reactors. Nuclear Engineering Division, U.S. Nuclear Regulatory Commission, U.S. Department of Energy.
- 1 2 Bonin, Bernhard; Klein, Etienne (2012). Le nucléaire expliqué par des physiciens.
- ↑ Bays SE, Ferrer RM, Pope MA, Forget B (February 2008). "Neutronic Assessment of Transmutation Target Compositions in Heterogeneous Sodium Fast Reactor Geometries" (PDF). Idaho National Laboratory, U.S. Department of Energy. INL/EXT-07-13643 Rev. 1.
- ↑ Plus radium (element 88). While actually a sub-actinide, it immediately precedes actinium (89) and follows a three-element gap of instability after polonium (84) where no isotopes have half-lives of at least four years (the longest-lived isotope in the gap is radon-222 with a half life of less than four days). Radium's longest lived isotope, at 1,600 years, thus merits the element's inclusion here.
- ↑ Specifically from thermal neutron fission of U-235, e.g. in a typical nuclear reactor.
- ↑ Milsted, J.; Friedman, A. M.; Stevens, C. M. (1965). "The alpha half-life of berkelium-247; a new long-lived isomer of berkelium-248". Nuclear Physics. 71 (2): 299. doi:10.1016/0029-5582(65)90719-4.
"The isotopic analyses disclosed a species of mass 248 in constant abundance in three samples analysed over a period of about 10 months. This was ascribed to an isomer of Bk248 with a half-life greater than 9 y. No growth of Cf248 was detected, and a lower limit for the β− half-life can be set at about 104 y. No alpha activity attributable to the new isomer has been detected; the alpha half-life is probably greater than 300 y." - ↑ This is the heaviest isotope with a half-life of at least four years before the "Sea of Instability".
- ↑ Excluding those "classically stable" isotopes with half-lives significantly in excess of 232Th; e.g., while 113mCd has a half-life of only fourteen years, that of 113Cd is nearly eight quadrillion years.
- ↑ Lineberry MJ, Allen TR (October 2002). "The Sodium-Cooled Fast Reactor (SFR)" (PDF). Argonne National Laboratory, US Department of Energy. ANL/NT/CP-108933.
External links
- Idaho National Laboratory Sodium-cooled Fast Reactor Fact Sheet
- Generation IV International Forum SFR website
- INL SFR workshop summary
- ALMR/PRISM
- ASME
- Richardson JH (November 17, 2009). "Meet the Man Who Could End Global Warming". Esquire.
... Eric Loewen is the evangelist of the sodium fast reactor, which burns nuclear waste, emits no CO2, ...