Stereolithography
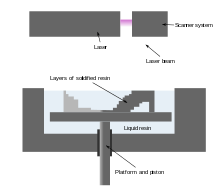
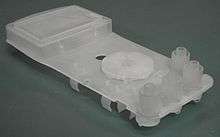
Stereolithography (SLA or SL; also known as stereolithography apparatus, optical fabrication, photo-solidification, or resin printing) is a form of 3-D printing technology used for creating models, prototypes, patterns, and production parts in a layer by layer fashion using photopolymerization, a process by which light causes chains of molecules to link together, forming polymers.[1] Those polymers then make up the body of a three-dimensional solid. Research in the area had been conducted during the 1970s, but the term was coined by Charles (Chuck) W. Hull in 1986 when he patented the process. He then set up 3D Systems Inc to commercialize his patent.[2][3]
History
Stereolithography or "SLA" printing is an early and widely used 3D printing technology. Also known as Rapid Prototyping, 3D printing was invented with the intent of allowing engineers to create prototypes of their designs in a more time effective manner.[3][4] The technology first appeared as early as the 1970s. Japanese researcher Dr. Hideo Kodama first invented the modern layered approach to stereolithography by using ultraviolet light to cure photosensitive polymers.[3] On July 16, 1984, three weeks before Chuck Hull filed his own patent, Alain Le Mehaute, Olivier de Witte and Jean Claude André filed a patent for the stereolithography process.[5] The French inventors' patent application was abandoned by the French General Electric Company (now Alcatel-Alsthom) and CILAS (The Laser Consortium). Le Mehaute believes that the abandonment reflects a problem with innovation in France.[6][7]
However, the term “stereolithography” was coined in 1986 by Chuck Hull.[1] Chuck Hull patented stereolithography as a method of creating 3D objects by successively "printing" thin layers of an object using a medium curable by ultraviolet light, starting from the bottom layer to the top layer. Hull's patent described a concentrated beam of ultraviolet light focused onto the surface of a vat filled with a liquid photopolymer. The UV light beam is focused onto the surface of the liquid photopolymer, creating each layer of the desired 3D object by means of crosslinking (or degrading a polymer). In 1986, Hull founded the world's first 3D printing company, 3D Systems Inc,[2][8][9] which is currently based in Rock Hill, SC.
Stereolithography's success in the automotive industry allowed 3D printing to achieve industry status and the technology continues to find innovative uses in many fields of study.[4][10] Attempts have been made to construct mathematical models of stereolithography processes and to design algorithms to determine whether a proposed object may be constructed using 3D printing.[11]
Technology
Stereolithography is an additive manufacturing process that works by focusing an ultraviolet (UV) laser on to a vat of photopolymer resin.[12] With the help of computer aided manufacturing or computer aided design software (CAM/CAD),[13] the UV laser is used to draw a pre-programmed design or shape on to the surface of the photopolymer vat. Because photopolymers are photosensitive under ultraviolet light, the resin is solidified and forms a single layer of the desired 3D object.[14] This process is repeated for each layer of the design until the 3D object is complete.
In models featuring an elevator apparatus, [15] an elevator platform descends a distance equal to the thickness of a single layer of the design (typically 0.05 mm to 0.15 mm) into the photopolymer vat. Then, a resin-filled blade sweeps across a cross section of the layer, re-coating it with fresh material.[15] The subsequent layer is traced, joining the previous layer. A complete 3D object can be formed using this process. Designs are then immersed in a chemical bath in order to remove any excess resin and cured in an ultraviolet oven.[15]
It is also possible to print objects "bottom up" by using a vat with a somewhat flexible, transparent bottom, and focusing the UV or deep-blue polymerization laser upward through the bottom of the vat.[16] For example, the Form 1 stereolithography machine starts a print by lowering the build platform to touch the bottom of the resin-filled vat, then moving upward the height of one layer. The UV laser then writes the bottom-most layer of the desired part upward through the transparent vat bottom, and the photopolymer hardens selectively where the laser strikes. Then the vat is "rocked", flexing and peeling the bottom of the vat away from the hardened photopolymer; the hardened material detaches from the bottom of the vat and stays attached to the rising build platform, and new liquid photopolymer flows in from the edges of the partially built part. The UV laser then writes the second-from-bottom layer and repeats the process. An advantage of this bottom-up mode is that the build volume can be much bigger than the vat itself, and only enough photopolymer is needed to keep the bottom of the build vat continuously full of photopolymer.
Stereolithography requires the use of supporting structures which attach to the elevator platform to prevent deflection due to gravity and to hold cross sections in place in order to resist lateral pressure from the resin-filled blade or retain newly created sections during the "vat rocking" of bottom-up printing. Supports are created automatically during the preparation of 3D Computer Aided Design models and can also be made manually.[15] With more expensive stereolithography models, these supports must be removed from the finished product manually.
Uses
Medical modeling
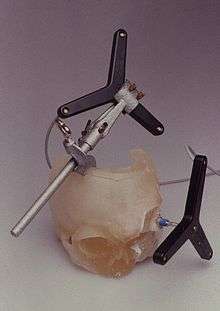
Stereolithographic models have been used in medicine since the 1990s,[17] for creating accurate 3D models of various anatomical regions of a patient, based on datasets from computer scans.[18] Medical modelling involves first acquiring a 3D CT, MRI, or other scan.[19] This data consists of a series of cross sectional images of the human anatomy. In these images different tissues show up as different levels of grey. Selecting a range of grey values enables specific tissues to be isolated. A region of interest is then selected and all the pixels connected to the target point within that grey value range are selected. This enables a specific organ to be selected. This process is referred to as segmentation. The segmented data may then be translated into a format suitable for stereolithography.[20] While stereolithography is normally accurate, the accuracy of a medical model depends on many factors, especially the operator performing the segmentation correctly. There are potential errors possible when making medical models using stereolithography but these can be avoided with practice and well trained operators.[21]
Stereolithographic models are used as an aid to diagnosis, preoperative planning and implant design and manufacture. This might involve for example planning and rehearsing osteotomies. Surgeons use models to help plan surgeries but prosthetists and technologists also use models as an aid to the design and manufacture of custom-fitting implants. Medical models are frequently used to help in the construction of Cranioplasty plates for example.
Prototyping
Stereolithography is often used for prototyping parts. For a relatively low price, Stereolithography can produce accurate prototypes, even of irregular shapes.[22] Businesses can use those prototypes to assess the design of their product or as publicity for the final product.[23]
Advantages and disadvantages
One of the advantages of stereolithography is its speed; functional parts can be manufactured within a day.[4] The length of time it takes to produce a single part depends upon the complexity of the design and the size. Printing time can last anywhere from hours to more than a day.[4] Many 3D printers can produce parts with a maximum size of approximately 50×50×60 cm (20×20×24 in) and some printers, such as the Mammoth stereolithography machine (which has a build platform of 210×70×80 cm),[24] are capable of producing single parts more than 2 meters in length. 3D printed prototypes and designs are strong enough to be machined and can also be used to make master patterns for injection molding, thermoforming, blow molding, and various metal casting processes.
Although stereolithography can be used to produce virtually any synthetic design,[13] it is often costly; common photopolymers can cost about $800 per gallon and SLA machines can cost $250,000.[25] Recently, public interest in 3D printing has inspired the design of several consumer SLA machines which can cost $3,500 or less, such as the Form 2 by Formlabs[16] or the Nobel 1.0 by XYZPrinting.[26] There has also been a reduction of the cost of photopolymer resins.
See also
References
- 1 2 U.S. Patent 4,575,330 (“Apparatus for Production of Three-Dimensional Objects by Stereolithography”)
- 1 2 Stereolithography/ 3D Printing/ Additive Fabrication
- 1 2 3 Gibson, Ian, and Jorge Bártolo, Paulo. “History of Stereolithography.” Stereolithography: Materials, Processes, and Applications. (2011): 41-43. Web. 7 October 2015.
- 1 2 3 4 Hull, Chuck. “On Stereolithography.” Virtual and Physical Prototyping. Vol 7. (2012): 177. Web. 11 Oct, 2015
- ↑ Jean-Claude, Andre. "Disdpositif pour realiser un modele de piece industrielle". National De La Propriete Industrielle.
- ↑ Moussion, Alexandre (2014). "Interview d'Alain Le Méhauté, l'un des pères de l'impression 3D". Primante 3D.
- ↑ Mendoza, Hannah Rose (May 15, 2015). "3dprint.com". Alain Le Méhauté, The Man Who Submitted Patent For SLA 3D Printing Before Chuck Hull.
- ↑ 3D Systems Inc Company Info
- ↑ What is Stereolithography?
- ↑ Jacobs, Paul F. “Introduction to Rapid Prototyping and Manufacturing.” Rapid Prototyping and Manufacturing: Fundamentals of Stereolithography. 1st Ed. (1992): 4-6. Web. 7 October 2015.
- ↑ B. Asberg, G. Blanco, P. Bose, J. Garcia-Lopez, M. Overmars, G. Toussaint, G. Wilfong and B. Zhu, "Feasibility of design in stereolithography," Algorithmica, Special Issue on Computational Geometry in Manufacturing, Vol. 19, No. 1/2, Sept/Oct, 1997, pp. 61–83.
- ↑ Crivello, James V., and Elsa Reichmanis. "Photopolymer Materials and Processes for Advanced Technologies." Chemistry of Materials Chem. Mater. 26.1 (2014): 533. Print.
- 1 2 Lipson, Hod, Francis C. Moon, Jimmy Hai, and Carlo Paventi. "3-D Printing the History of Mechanisms." Journal of Mechanical Design J. Mech. Des. (2004): 1029-033. Print.
- ↑ Fouassier, J. P. "Photopolymerization Reactions." The Wiley Database of Polymer Properties 3 (2003): 25. Print.
- 1 2 3 4 "Stereolithography (SLA)". www.amtech-rp.co.uk. Retrieved 2015-11-16.
- 1 2 Ngo, Dong. "Formlabs Form 2 3D Printer review: An excellent 3D printer for a hefty price". CNET. Retrieved 3 August 2016.
More specifically, as the print platform lowers itself into the resin glass tank, an ultraviolet laser light, from underneath the see-through tank, shines on it. (For this reason, SLA is sometimes called the laser 3D-printing technology.) Exposed to the laser light, the resin cures, solidifies and sticks to the platform. As more resin is exposed to the laser light, the pattern is created and joins the layer above. As more and more layers are being created, the build platform slowly -- very slowly -- moves upward, finally pulling the entire object out of the tank as the print process is finished.
- ↑ Klimek, L; Klein HM; Schneider W; Mosges R; Schmelzer B; Voy ED (1993). "Stereolithographic modelling for reconstructive head surgery". Acta Oto-Rhino-Laryngologica Belgica. 47 (3): 329–34.
- ↑ Bouyssie, JF; Bouyssie S; Sharrock P; Duran D (1997). "Stereolithographic models derived from x-ray computed tomography. Reproduction accuracy". Surgical & Radiologic Anatomy. 19 (3): 193–9.
- ↑ Winder, RJ; Bibb, R (2009). "A Review of the Issues Surrounding Three-Dimensional Computed Tomography for Medical Modelling using Rapid Prototyping Techniques". Radiography. 16: 78–83. doi:10.1016/j.radi.2009.10.005.
- ↑ Bibb, Richard (2006). Medical Modelling: the application of advanced design and development technologies in medicine. Cambridge: Woodhead Publishing Ltd. ISBN 1-84569-138-5.
- ↑ Winder, RJ; Bibb, R (2005). "Medical Rapid Prototyping Technologies: State of the Art and Current Limitations for Application in Oral and Maxillofacial Surgery". Journal of Oral and Maxillofacial Surgery. 63 (7): 1006–15. doi:10.1016/j.joms.2005.03.016.
- ↑ Palermo, Elizabeth (16 July 2013). "What is Stereolithography?". Live Science. Purch Group. Retrieved 7 October 2016.
- ↑ "Applications of SLA". Stereolithography. Retrieved 7 October 2016.
- ↑ Mammoth stereolithography: Technical specifications. materialise.com
- ↑ Brain, Marshall. "How Stereolithography 3-D Layering Works". Howstuffworks. Infospace LLC. Retrieved 17 December 2015.
- ↑ Ngo, Dong. "XYZprinting Nobel 1.0 3D Printer review: Highly detailed prints at a high cost". CNET. Retrieved 3 August 2016.
Notes
- Kalpakjian, Serope and Steven R. Schmid. Manufacturing Engineering and Technology 5th edition. Ch. 20 (pp. 586–587 Pearson Prentice Hall. Upper Saddle River NJ, 2006.
External links
- Rapid Prototyping and Stereolithography animation – Animation demonstrates stereolithography and the actions of an SL machine
- Video of micro-stereolithography for biomedical applications