Gene drive
In genetics, gene drive is a technique that promotes the inheritance of a particular gene to increase its prevalence in a population.[1] The term is also used for specific genetic elements (i.e. a piece of DNA) that can implement the technique.
Applications of gene drive include preventing the spread of insects that carry diseases (in particular, mosquitoes that transmit malaria, dengue, and zika), to control invasive species, or to eliminate herbicide or pesticide resistance.[1][2][3] The technique can be used for adding, disrupting, or modifying genes, such as to cause a crash in the populations of a disease vector by reducing their reproductive capacity.[2][4]
Several molecular mechanisms can mediate gene drive.[5] Naturally occurring gene drive mechanisms arise when alleles evolve molecular mechanisms that give them a transmission chance greater than the normal 50%. Synthetic genetic modules with similar properties have been developed as a technique for genome editing of laboratory populations. This entry focuses on endonuclease-based gene drive, the most versatile and actively developing molecular backend for synthetic gene drives. Since gene drives function only in sexually reproducing species, they cannot be used to engineer populations of viruses or bacteria.
Because it is a way to artificially bias inheritance of desired genes, gene drive constitutes a major change in biotechnology. The potential impact of releasing gene drives in the wild raises major bioethical concerns regarding their development and management.
Mechanism
In sexually-reproducing species, most genes are present in two copies (which can be different alleles or not), each of which has 50% chance of being inherited. For a particular allele to spread through a large population, it must increase the fitness of each individual. However, some alleles have evolved molecular mechanisms that confer them greater transmission chance than the normal 50%. This allows them to spread through a population even if they reduce the fitness of each individual organism. By similarly biasing the inheritance of particular altered genes, synthetic gene drives might be used to spread alterations through wild populations.[2][4]
Molecular mechanisms
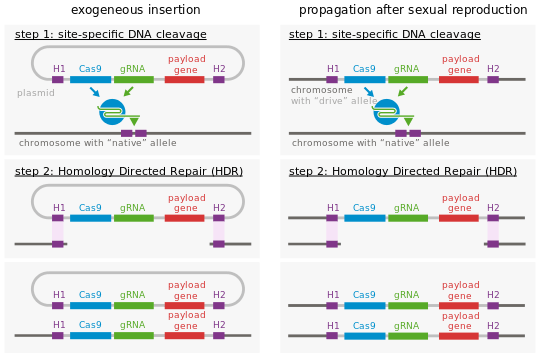
At the molecular level, endonuclease gene drives work by cutting chromosomes that do not encode the drive at a specific site, inducing the cell to repair the damage by copying the drive sequence onto the damaged chromosome. This is derived from genome editing techniques and similarly relies on the fact that double strand breaks are most frequently repaired by homologous recombination if a template is present, and less often by non-homologous end joining. The cell then has two copies of the drive sequence. To achieve this behavior, endonuclease gene drives consist of two nested elements:
- either a homing endonuclease or a RNA-guided endonuclease (e.g. Cas9 or Cpf1) and its guide RNA, that cuts the target sequence in recipient cells
- a template sequence used by the DNA repair machinery after the target sequence is cut. To achieve the self propagating nature of gene drives, this repair template contains at least the endonuclease sequence. Because the template must be used to repair a double-strand break at the cutting site, its sides are homologous to the sequences that are adjacent to the cutting site in the host genome. By targeting the gene drive to a gene coding sequence, this gene will be inactivated; additional sequences can be introduced in the gene drive to encode new functions.
As a result, the gene drive insertion in the genome will re-occur in each organism that inherits one copy of the modification and one copy of the wild-type gene. If the gene drive is already present in the egg cell (e.g. when received from one parent), all the gametes of the individual will carry the gene drive (instead of 50% in the case of a normal gene).
Spreading in the population
Since it can never more than double in frequency with each generation, a gene drive introduced in a single individual typically requires dozens of generations to affect a substantial fraction of a population. Alternatively, releasing drive-containing organisms in sufficient numbers can affect the rest within a few generations; for instance, by introducing it in every thousandth individual, it takes only 12-15 generations to be present in all individuals.[6] Whether a gene drive will ultimately become fixed in a population and at which speed depends on its effect on individuals fitness, on the rate of allele conversion, and on the population structure. In a well mixed population and with realistic allele conversion frequencies (≈90%), population genetics predicts that gene drives get fixed for selection coefficient smaller than 0.3;[6] in other words, gene drives can be used not only to spread beneficial genetic modifications, but also detrimental ones as long the reproductive success is not reduced by more than 30%. This is a great contrast with normal genes, which can only spread in large populations if they are beneficial.
Applications and technical limitations
Applications
Gene drives have two main classes of applications, which, although based on the same technology, have implications of different significance:
- introduce a genetic modification in laboratory populations; once a strain or a line carrying the gene drive has been produced, the drive can be passed on to any other line simply by mating. Here the gene drive is used to achieve much more easily a task that could be accomplished with other techniques. It requires reinforced confinement of the lab populations to prevent an accidental release of the gene drive into the wild.
- introduce a genetic modification in wild populations. In contrast with the former, gene drives here constitute a major development and open the door to previously unattainable changes. This raises major ethical issues.
Because of the unprecedented potential of gene drives, safeguard mechanisms have been proposed and tested.[7]
Technical limitations
Because gene drives propagate by replacing other alleles that contain a cutting site and the corresponding homologies, their application is limited to sexually reproducing species (because they are diploid and alleles are mixed at each generation). As a side effect, inbreeding could in principle be selected as an escape mechanism, but the extent to which this can happen in practice is difficult to evaluate.[8]
Due to the number of generations required for a gene drive to spread in an entire population, it may require under a year for some invertebrates, but centuries for organisms with years-long intervals between birth and sexual maturity, such as humans.[9] Hence this technology is of most use in fast-reproducing species.
Issues
Issues that researchers have highlighted include:[10]
- Mutations—It is possible that a mutation could happen mid-drive, which has the potential to allow unwanted traits to "ride along" on the spreading drive.
- Escape—Cross-breeding or gene flow potentially allow a drive to move beyond its target population.
- Ecological impacts—Even when new traits' direct impact on a target is understood, the drive may have side effects on the surroundings.
There are bioethics concerns as well, as the gene drive is a very powerful tool.[11]
In December 2015, scientists of major world academies called for a moratorium on inheritable human genome edits that would be passed on in pregnancies, including those related to CRISPR-Cas9 technologies,[12] but supported continued basic research and gene editing that would not affect future generations.[13] In February 2016, British scientists were given permission by regulators to genetically modify human embryos by using CRISPR-Cas9 and related techniques on condition that the embryos were destroyed in seven days.[14][15] In June 2016, the US National Academies of Sciences, Engineering, and Medicine released a report on their "Recommendations for Responsible Conduct" of gene drives.[16]
History
Austin Burt, an evolutionary geneticist at Imperial College London, first outlined the possibility of building gene drives based on natural "selfish" homing endonuclease genes in 2003.[4] Researchers had already shown that these “selfish” genes could spread rapidly through successive generations. Burt suggested that gene drives might be used to prevent a mosquito population from transmitting the malaria parasite or crash a mosquito population. Gene drives based on homing endonucleases have been demonstrated in the laboratory in transgenic populations of mosquitoes[17] and fruit flies.[18][19] However, homing endonucleases are sequence-specific. Since altering their specificity to target other sequences of interest remains a major challenge,[5] the possible applications of gene drive remained limited until the discovery of CRISPR and the associated RNA-guided endonucleases such as Cas9 and Cpf1.
In August 2016 the U.S. Food And Drug Administration (FDA) issued a “Finding of No Significant Impact" (FONSI) to biotech company Oxitec's plan to release genetically modified male Aedes Aegypti (mosquitoes) into the Florida Keys. The intent was to stop the spread of mosquito-borne diseases, including Zika. The modification adds a gene that kills their offspring before they reach reproductive age. Oxitec still needs approval from the Florida Keys Mosquito Control District before releasing any insects.[20]
The Bill and Melinda Gates Foundation has invested $75 million in gene drive technology. The foundation originally estimated the technology to be ready for field use by 2029 somewhere in Africa. However, Gates in 2016 changed this estimate to instead happen some time within the next two years.[21]
CRISPR/Cas9
CRISPR/Cas9[22] is a DNA cutting method that has made genetic engineering faster, easier, and more efficient since 2013.[23] The approach involves expressing the RNA-guided Cas9 endonuclease along with guide RNAs directing it to a particular sequence to be edited. When Cas9 cuts the target sequence, the cell often repairs the damage by replacing the original sequence with homologous DNA. By introducing an additional template with appropriate homologies, Cas9 can be used to delete, add, or modify genes in an unprecedentedly simple manner. As of 2014, it had successfully been tested in cells of 20 species, including humans.[2] In many of these species, the edits modified their germline, allowing them to be inherited.
Esvelt and coworkers first suggested in 2014 that CRISPR/Cas9 might be used to build endonuclease gene drives.[2] In 2015 researchers published successful engineering of CRISPR-based gene drives in Saccharomyces[7], Drosophila[24] and mosquitoes.[25][26] All four studies demonstrated extremely efficient inheritance distortion over successive generations, with one study demonstrating the spread of a gene drive into naïve laboratory populations.[26] Drive-resistant alleles are expected to arise for each of the described gene drives, however this can be delayed or prevented by targeting highly conserved sites at which resistance is expected to have a severe fitness cost.
Because of CRISPR/Cas9's targeting flexibility, the derived gene drives could theoretically be used to engineer almost any trait. Unlike previous designs, they could be tailored to block the evolution of drive resistance in the target population by targeting multiple sequences within appropriate genes. CRISPR/Cas9 could also permit a variety of gene drive architectures intended to control rather than crash populations. Noticeably, RNA-guided gene drives could be designed with other RNA-guided endonuclease such as CRISPR/Cpf1.
Applications to wild populations
Disease vector species
One possible application is to genetically modify mosquitoes and other disease vectors so they cannot transmit diseases such as malaria and dengue fever. In June 2014, the World Health Organization (WHO) Special Programme for Research and Training in Tropical Diseases[27] issued guidelines[28] for evaluating genetically modified mosquitoes. In 2013 the European Food Safety Authority issued a protocol[29] for environmental assessments of all genetically modified organisms. Researchers believe that by applying the new technique to just 1% of the wild population of mosquitoes, they can eradicate malaria within a year.[30]
Invasive species
Gene drive could be used to eliminate invasive species and has, for example, been proposed as a way to eliminate invasive species in New Zealand;[31] in response others have raised the standard objections to the use of gene drive.[32]
See also
- Biological machines
- Cas9
- CRISPR/Cpf1
- Meiotic drive
- Genome editing
- Population control
- Synthetic biology
References
- 1 2 "U.S. researchers call for greater oversight of powerful genetic technology | Science/AAAS | News". News.sciencemag.org. Retrieved 2014-07-18.
- 1 2 3 4 5 Esvelt, Kevin M; Smidler, Andrea L; Catteruccia, Flaminia; Church, George M (July 2014). "Concerning RNA-guided gene drives for the alteration of wild populations". eLife. 3: e03401. doi:10.7554/eLife.03401. PMID 25035423.
- ↑ Benedict, M.; D'Abbs, P.; Dobson, S.; Gottlieb, M.; Harrington, L.; Higgs, S.; James, A.; James, S.; Knols, B.; Lavery, J.; O'Neill, S.; Scott, T.; Takken, W.; Toure., Y. (April 2008). "Vector-Borne and Zoonotic Diseases". Vector-Borne and Zoonotic Diseases. 8 (2): 127–166. doi:10.1089/vbz.2007.0273. PMID 18452399.
- 1 2 3 Burt, A. (2003). "Site-specific selfish genes as tools for the control and genetic engineering of natural populations". Proceedings of the Royal Society B: Biological Sciences. 270 (1518): 921– 928. doi:10.1098/rspb.2002.2319.
- 1 2 Champer, Jackson; Buchman, Anna; Akbari, Omar S. "Cheating evolution: engineering gene drives to manipulate the fate of wild populations". Nature Reviews Genetics. 17 (3): 146–159. doi:10.1038/nrg.2015.34.
- 1 2 Unckless, Robert L.; Messer, Philipp W.; Connallon, Tim; Clark, Andrew G. (2015-10-01). "Modeling the Manipulation of Natural Populations by the Mutagenic Chain Reaction". Genetics. 201 (2): 425–431. doi:10.1534/genetics.115.177592. ISSN 0016-6731. PMC 4596658
. PMID 26232409.
- 1 2 Dicarlo, J. E.; Chavez, A.; Dietz, S. L.; Esvelt, K. M.; Church, G. M. (2015). "RNA-guided gene drives can efficiently and reversibly bias inheritance in wild yeast". doi:10.1101/013896.
- ↑ Bull, James J. (2016-04-02). "Lethal Gene Drive Selects Escape through Inbreeding". bioRxiv: 046847. doi:10.1101/046847.
- ↑ Oye, Kenneth A.; Esvelt, Kevin; Appleton, Evan; Catteruccia, Flaminia; Church, George; Kuiken, Todd; Lightfoot, Shlomiya Bar-Yam; McNamara, Julie; Smidler, Andrea (2014-08-08). "Regulating gene drives". Science. 345 (6197): 626–628. doi:10.1126/science.1254287. ISSN 0036-8075. PMID 25035410.
- ↑ Drinkwater, Kelly; Kuiken, Todd; Lightfoot, Shlomiya; McNamara, Julie; Oye, Kenneth. "CREATING A RESEARCH AGENDA FOR THE ECOLOGICAL IMPLICATIONS OF SYNTHETIC BIOLOGY" (PDF). The Wilson Center and the Massachusetts Institute of Technology Program on Emerging Technologies.
- ↑ "Genetically Engineering Almost Anything". PBS. 17 Jul 2014.“I don’t care if it’s a weed or a blight, people still are going to say this is way too massive a genetic engineering project,” [bioethicist] Caplan says. “Secondly, it’s altering things that are inherited, and that’s always been a bright line for genetic engineering.”
- ↑ Wade, Nicholas (3 December 2015). "Scientists Place Moratorium on Edits to Human Genome That Could Be Inherited". New York Times. Retrieved 3 December 2015.
- ↑ Huffaker, Sandy (9 December 2015). "Geneticists vote to allow gene editing of human embryos". New Scientist. Retrieved 18 March 2016.
- ↑ Gallagher, James (1 February 2016). "Scientists get 'gene editing' go-ahead". BBC News. BBC. Retrieved 1 February 2016.
- ↑ Cheng, Maria (1 February 2016). "Britain approves controversial gene-editing technique". AP News. Retrieved 1 February 2016.
- ↑ "Gene Drive Research in Non-Human Organisms: Recommendations for Responsible Conduct". National Academies of Sciences, Engineering, and Medicine. June 8, 2016. Retrieved June 9, 2016.
- ↑ Windbichler, N.; Menichelli, M.; Papathanos, P. A.; Thyme, S. B.; Li, H.; Ulge, U. Y.; Hovde, B. T.; Baker, D.; Monnat Jr, R. J.; Burt, A.; Crisanti, A. (2011). "A synthetic homing endonuclease-based gene drive system in the human malaria mosquito". Nature. 473 (7346): 212–215. doi:10.1038/nature09937. PMC 3093433
. PMID 21508956.
- ↑ Chan, Y.-S. (2011). "Insect Population Control by Homing Endonuclease-Based Gene Drive: An Evaluation in Drosophila melanogaster". Genetics. 188 (1): 33–44. doi:10.1534/genetics.111.127506.
- ↑ Chan, Yuk-Sang (2013). "Optimising Homing Endonuclease Gene Drive Performance in a Semi-Refractory Species: The Drosophila melanogaster Experience". PLoS ONE. 8 (1): e54130. doi:10.1371/journal.pone.0054130.
- ↑ "FDA Approves Genetically Modified Mosquitoes For Release In Florida". Retrieved 2016-08-07.
- ↑ Regalado, Antonio. "Bill Gates doubles his bet on wiping out mosquitoes with gene editing". Retrieved 2016-09-20.
- ↑ Elizabeth Pennisi (2013-08-23). "The CRISPR Craze". Sciencemag.org. Retrieved 2014-07-18.
- ↑ Pollack, Andrew (May 11, 2015). "Jennifer Doudna, a Pioneer Who Helped Simplify Genome Editing". New York Times. Retrieved May 12, 2015.
- ↑ Gantz, V. M.; Bier, E. (2015). "The mutagenic chain reaction: A method for converting heterozygous to homozygous mutations". Science. 348: 442–444. doi:10.1126/science.aaa5945.
- ↑ Gantz, Valentino M.; Jasinskiene, Nijole; Tatarenkova, Olga; Fazekas, Aniko; Macias, Vanessa M.; Bier, Ethan; James, Anthony A. (2015-12-08). "Highly efficient Cas9-mediated gene drive for population modification of the malaria vector mosquito Anopheles stephensi". Proceedings of the National Academy of Sciences. 112 (49): E6736–E6743. doi:10.1073/pnas.1521077112. ISSN 0027-8424. PMID 26598698.
- 1 2 Hammond, Andrew; Galizi, Roberto; Kyrou, Kyros; Simoni, Alekos; Siniscalchi, Carla; Katsanos, Dimitris; Gribble, Matthew; Baker, Dean; Marois, Eric (2015-12-07). "A CRISPR-Cas9 gene drive system targeting female reproduction in the malaria mosquito vector Anopheles gambiae". Nature Biotechnology. advance online publication. doi:10.1038/nbt.3439. ISSN 1546-1696.
- ↑ "TDR | About us". Who.int. Retrieved 2014-07-18.
- ↑ "TDR | A new framework for evaluating genetically modified mosquitoes". Who.int. 2014-06-26. Retrieved 2014-07-18.
- ↑ "EFSA - Guidance of the GMO Panel: Guidance Document on the ERA of GM animals". Efsa.europa.eu. doi:10.2903/j.efsa.2013.3200. Retrieved 2014-07-18.
- ↑ https://www.youtube.com/watch?v=OI_OhvOumT0 Gene editing can now change an entire species -- forever | Jennifer Kahn
- ↑ Kalmakoff, James (11 October 2016). "CRISPR for pest-free NZ". Retrieved 19 October 2016.
- ↑ Campbell, Colin (17 October 2016). "Risks may accompany gene drive technology". Otago Daily Times. Retrieved 19 October 2016.
External links
- Esvelt, Kevin. "Gene Drives for the Alteration of Wild Populations". Retrieved 11 August 2014.
- "Gene Drive from Harvard's Wyss Institute". Wyss Institute. 2014-07-17. Retrieved 2014-08-11.
- De Chant, Tim (July 17, 2014). "Genetically Engineering Almost Anything". NOVA. Retrieved 11 August 2014.
- Johnson, Carolyn (July 17, 2014). "Harvard scientists want gene-manipulation debate". National Geographic. Retrieved 11 August 2014.
- Langin, Katie (July 17, 2014). "Genetic Engineering to the Rescue Against Invasive Species?". National Geographic. Retrieved 11 August 2014.
- Zimmer, Carl (July 17, 2014). "A Call to Fight Malaria One Mosquito at a Time by Altering DNA". The New York Times. Retrieved 20 July 2014.
- "The age of the red pen". The Economist. August 22, 2015. ISSN 0013-0613. Retrieved 2015-08-25.
- "The most selfish genes". The Economist. August 22, 2015. ISSN 0013-0613. Retrieved 2015-08-25.