Interplanetary contamination
Interplanetary contamination refers to biological contamination of a planetary body by a space probe or spacecraft, either deliberate or unintentional.
There are two types of interplanetary contamination:
- Forward contamination is the transfer of life and other forms of contamination from Earth to another celestial body.
- Back contamination is the introduction of extraterrestrial organisms and other forms of contamination into Earth's biosphere. It also covers infection of humans and human habitats in space and on other celestial bodies by extraterrestrial organisms, if such habitats exist.
The main focus is on microbial life and on potentially invasive species. Non-biological forms of contamination have also been considered, including contamination of sensitive deposits (such as lunar polar ice deposits) of scientific interest by rocket exhausts. In the case of back contamination, multicellular life is thought unlikely but has not been ruled out. In the case of forward contamination, contamination by multicellular life (e.g. lichens) is unlikely to occur for robotic missions, but it becomes a consideration in human missions.
Current space missions are governed by the Outer Space Treaty and the COSPAR guidelines for planetary protection. Forward contamination is prevented primarily by sterilizing the spacecraft. In the case of backward contamination, however, the aim of the mission is to return biological material from foreign planets to Earth if such exists, and sterilization of the samples would make them of much less interest. So, back contamination would be prevented mainly by containment, and breaking the chain of contact between the foreign planet of origin and Earth. It would also require quarantine procedures for the materials and for anyone who comes into contact with them.
Vulnerability of the Solar System to contamination
Most of the solar system appears hostile to life as we know it. No extraterrestrial life has ever been discovered, but there are several locations outside Earth where life could possibly exist, have existed, or thrive if introduced.
If extraterrestrial life exists, it may be vulnerable to interplanetary contamination by foreign micro-organisms. Some extremophiles are able to survive space travel to another planet, and foreign life could possibly be introduced by spacecraft from Earth, for example. If extraterrestrial life does not exist, but could potentially thrive in a given location, the site is also considered vulnerable to contamination. In this case, the introduction of life could transform the location from its current pristine state.
Locations within the Solar System where life might exist today include the ocean of liquid water beneath the icy surface of Europa, the interior of Enceladus which is currently sending water into space, and the possible ocean of liquid water which some astronomers believe might exist beneath the surface of Titan (its surface has oceans of liquid ethane / methane, but it may also have liquid water below the surface and ice volcanoes).[1][2]
Mars
There is enough evidence to suggest that Mars once offered habitable conditions for microbial life.[3][4] It is therefore possible that life may have existed on Mars, although no evidence has been found, and it is even possible that life still exists today in the subsurface and shallow subsurface (top few cm).[5][6][7][8][9][10][11]
It is currently believed that there are many dormant micro-organisms from Earth on the surface of Mars. For instance, some may be protected within the Martian rovers on the planet.[12][13] In that sense, Mars may have already been interplanetarily contaminated.
However, it is also believed that this contamination is only by dormant micro-organisms that have not yet encountered conditions permitting them to metabolize and reproduce.[14][15] If so, then a future human colonization may perhaps be biologically reversible. If needed, it may be possible to remove all the life we brought to Mars and return the planet to its previous biologically pristine state. This is a suggestion made by Christopher McKay.[16]
Several theoretical and simulatory studies have shown that some lifeforms from Earth can potentially survive under Martian conditions. In particular, certain lichens from the arctic permafrost are able to photosynthesize and grow in the absence of any liquid water, simply by using the humidity from the atmosphere. They are also highly tolerant of UV radiation, using melanin and other more specialized chemicals to protect their cells. The air on Mars approaches 100% humidity in evening and morning, and at those times frosts form on the surface. These are also conditions which might make it possible for lichens to survive there.
On 26 April 2012, scientists reported that lichen survived and showed remarkable results on the adaptation capacity of photosynthetic activity within the simulation time of 34 days under Martian conditions in the Mars Simulation Laboratory (MSL) maintained by the German Aerospace Center (DLR).[17][18]
Other studies have suggested the potential for life to survive using deliquescing salts. These, similarly to the lichens, use the humidity of the atmosphere. If the mixture of salts is right, they may support liquid water at times of high atmospheric humidity, with salts dilute enough to be capable of supporting life. This water would be warm enough (warmer than about -25 °C) but cold enough so that it doesn't immediately evaporate in the thin Martian atmosphere. The salts and perchlorates required are known to exist on Mars, though whether they occur in the correct mixtures to support these marginally habitable deliquescing droplets and thin layers of salty brines is unknown.
Evidence for possible life-harbouring sites outside Earth
Enceladus, Europa, and Mars show the best evidence for hospitable habitats, mainly due to the possibility of their hosting liquid water and organic compounds.
Enceladus
The Cassini spacecraft has directly sampled the plumes escaping from Enceladus. Measured data indicates that these geysers are made primarily of salt rich particles with an 'ocean-like' composition, which is believed to originate from a subsurface ocean of liquid saltwater, rather than from the moon's icy surface.[19] Data from the geyser flythroughs also indicate the presence of basic organic chemicals in the plumes. Heat scans of Enceladus' surface also indicate warmer temperatures around the fissures where the geysers originate from, with temperatures reaching -93 °C (-135 °F), which is 115 °C (207 °F) warmer than the other regions on the moon's surface.[20]
Europa
Europa has much indirect evidence for its sub-surface ocean. Models of how Europa is affected by Tidal heating require a subsurface layer of liquid water or warm ice in order to accurately reproduce the linear fracturing of the surface. Indeed, observations by the Galileo spacecraft of how Europa's magnetic field interacts with Jupiter's field strengthens the case for a liquid, rather than solid, layer; an electrically conductive fluid deep within Europa would explain these results.[21] Observations from the Hubble Space Telescope in December 2012 appear to show an ice plume spouting from Europa's surface,[22] which would immensely strengthen the case for a liquid subsurface ocean. As was the case for Enceladus, vapour geysers would allow for easy sampling of the liquid layer.[23] Unfortunately, there appears to be little evidence that geysering is a frequent event on Europa due to the lack of water in the space near Europa.[24]
Mars
There is strong evidence from multiple Martian missions for liquid water on Mars. There is good indirect isotope evidence from the Phoenix lander which shows that some liquid on the surface, likely to be water, has exchanged oxygen atoms with the CO2 in the atmosphere in the recent geological past. This evidence is not yet sufficient to determine whether this is due to intermittent flooding or flows, such as after geothermal or volcanic activity or meteorite impacts, or whether the liquid water is present every year, as in some of the recent suggestions for shallow subsurface habitats.[25] The Mars Reconnaissance Orbiter's imaging spectrometer has discovered the presence of hydrated salts in recurrent slope lineae – dark downhill flows which darken during warm seasons and fade during cold seasons. The seasonal changes of these flows are highly indicative of shallow subsurface movement of brine.[26]
Dissenting views on vulnerability of the Solar System
Even though many scientists would say that there is a possibility that environments hospitable to life exist, there are dissenting views about whether it would make any difference if microbes from Earth are introduced to them.
In a 2013 paper in Nature Geoscience, "The Overprotection of Mars", Alberto Fairén and Dirk Schulze-Makuch suggested that we no longer need to protect Mars, arguing that if life can get to Mars on our spacecraft, then Earth life has probably already reached it on meteorites.[27] This was rebutted in a follow-up article in the same journal, "Appropriate Protection of Mars", by the current and previous planetary-protection officers Catherine Conley and John Rummel.[28][29]
Consequences
There are multiple consequences for both forward- and back-contamination.
- If a planet becomes contaminated by Earth life, it might then be difficult to tell whether any lifeforms discovered originated there or came from Earth.[30] Furthermore, the organic chemicals produced by the introduced life would confuse sensitive searches for biosignatures of living or ancient native life.
For instance, the astrobionibbler[31] is a sensitive tool proposed for Mars that would be able to detect a single amino acid in a gram of sample. If the sample is already contaminated by Earth life, then the discovery of an amino acid in it would be of considerably less interest than if it were uncontaminated.
The same applies to other more complex biosignatures. Searching for biological remains of the precursors to life, whether ancient or currently existing, would be far easier on an uncontaminated planet. This would similarly apply to searches for DNA sequences on Mars. Life on other planets could have a common origin with Earth life, since in the early Solar System there was much exchange of material between the planets which could have transferred life as well. If so it might be based on DNA too.
Most species of micro-organism on Earth are not yet well understood or DNA sequenced. This particularly applies to the uncultivable archaea, and the majority of micro-organisms are uncultivable, and so are hard to study. This can be either because they depend on the presence of other micro-organisms, or are slow growing, or depend on other conditions not yet well understood. In typical habitats, 99% of micro-organisms are uncultivable.[32]
This is true of species detected on spacecraft in clean rooms. The majority of the species detected are not well understood or characterized and can't be cultivated, and are known only from DNA fragments.[33]
On a contaminated planet, it might be hard to distinguish the DNA of extraterrestrial life from the DNA of life brought to the planet by our spacecraft, because of our incomplete knowledge of the DNA sequences of Earth micro-organisms.
- Invasive species could out compete native life or consume it, if there is life on the planet.[34]
One argument against this is that the native life would be more adapted to the conditions there. However, we have experience on Earth that species moved from one continent to another may be able to outcompete the native life adapted to that continent; rabbits introduced to Australia are able to outcompete the marsupials already there. The same may be true for micro-organisms introduced to other planets.[34] Additionally, evolutionary processes on Earth might have explored biological pathways not explored by life on other planets and so be able to out compete it. The same is also possible the other way around for back contamination.
- Introduced Earth life could contaminate resources of value for future human missions, e.g. contaminate possible sources of water.[35]
Current preventive methods
Forward contamination is prevented by sterilizing rovers sent to sensitive areas of the Solar System. Missions are classified depending on whether their destinations are of interest for the search for life, and whether there is any chance that life could reproduce there.
NASA made these policies official with the issuing of Management Manual NMI-4-4-1, NASA Unmanned Spacecraft Decontamination Policy on September 9, 1963.[36] Prior to NMI-4-4-1 the same sterilization requirements were required on all outgoing spacecraft regardless of their target. Difficulties in the sterilization of Ranger probes sent to the Moon are the primary reasons for NASA's change to a target-by-target basis in assessing the likelihood forward contamination.
Some destinations such as Mercury need no precautions at all. Others such as the Moon require documentation but nothing more, while destinations such as Mars require sterilization of the rovers sent there. For the details, see Planetary protection.
Backward contamination would be prevented by containment or quarantine; however, there have been no sample returns thought to have any possibility of a back contamination risk since the Apollo missions. The Apollo regulations have been rescinded and new regulations have yet to be developed, see Precautions suggested for sample return
Human colonization challenges
Human colonization is of particular concern for interplanetary contamination.
The main challenges are:
- the impossibility to sterilize human missions to the same level as a robotic spacecraft. Therefore, the chance of forward contamination must be higher than for a robotic mission.[37]
Humans are typically host to a hundred trillion micro-organisms in ten thousand species in the human microbiome which can't be removed while preserving the life of the human. Containment seems the only option, but effective containment to the same standard as a robotic rover appears difficult to achieve with present-day technology. In particular, adequate containment in the event of a hard landing is a major challenge.
- Human explorers' potential vulnerability to back contamination on the planet if any native life is hazardous to humans directly or indirectly.[38]
- Contamination of resources on the planet - particularly the water supply - by Earth micro-organisms, which could have a direct effect on the human colonization[35]
The Moon as a testbed
The Moon has been suggested as a testbed for Planetary protection for human missions in space. Currently the Moon has no contamination restrictions because it's considered to be "not of interest" for prebiotic chemistry and origins of life. It could be used to test new technology to protect sites in the Solar System, and astronauts, from forward and backward contamination. Analysis of the contamination left by the Apollo astronauts could also yield useful ground truth for planetary protection models.[39][40]
Non-contaminating exploration methods
Robotic exploration, telerobotics and telepresence give a method of exploration of a planet that do not have the same issues of forward and backward contamination. This could be of special interest during early stages when the celestial body is not yet well understood.
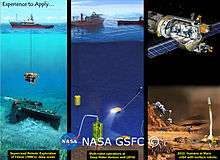
Michael Meltzer in his "When Biospheres Collide" - a history of NASA'S Planetary Protection Programs" wrote:[37]
"One of the most reliable ways to reduce the risk of forward contamination during visits to extraterrestrial bodies is to make those visits only with robotic spacecraft. Sending a person to Mars would be, for some observers, more exciting. But in the view of much of the space science community, robotic missions are the way to accomplish the maximum amount of scientific inquiry since valuable fuel and shipboard power do not have to be expended in transporting and operating the equipment to keep a human crew alive and healthy. And very important to planetary protection goals, robotic craft can be thoroughly sterilized, while humans cannot. Such a difference can be critical in protecting sensitive targets, such as the special regions of Mars, from forward contamination.
"Perhaps a change in the public's perspective as to just what today's robotic missions really are would be helpful in deciding what types of missions are important to implement. In the opinion of Terence Johnson, who has played a major role in many of NASA's robotic missions, including serving as the project scientist for the Galileo mission and the planned Europa Orbiter mission, the term "robotic exploration" misses the point. NASA is actually conducting human exploration on these projects. The mission crews that sit in the control panel at JPL, "as well as everyone else who can log on to the Internet" can observe in near real-time what is going on. The spacecraft instruments, in other words, are becoming more like collective sense organs for humankind. Thus, according to Johnson, when NASA conducts it's so-called robotic missions, people all around the world are really "all standing on the bridge of Starship Enterprise". The question must thus be asked, when, if ever, is it necessary for the good of humankind to send people rather than increasingly sophisticated robots to explore other worlds"
Humans in close orbit around the planet could control equipment on the surface in real time via telepresence, so bringing many of the benefits of a surface mission, without its associated increased forward and backward contamination risks.[41][42][43]
In site resource utilization is possible in orbital missions as for surface missions e.g. using resources mined from Deimos in the case of Mars.
Back contamination issues
Since the Moon is now generally considered to be free from life, the most likely source of contamination is from Mars during either a Mars sample return or as a result of colonization of Mars.
There are no immediate plans for a Mars sample return, but it remains a high priority for NASA and the ESA because of its great potential biological interest. The European Space Foundation report cites many advantages of a Mars sample return. In particular, it would permit extensive analysis with any of the equipment available on Earth, without the size and weight constraints for instruments sent to Mars on rovers. These analyses could also be carried out without the communication delays for experiments carried out on Martian rovers. It would also make it possible to repeat experiments in multiple laboratories with different instruments to confirm key results.[44]
Carl Sagan was first to raise and publicise back contamination issues that might follow from a Mars sample return. In Cosmic Connection (1973) he writes:
Precisely because Mars is an environment of great potential biological interest, it is possible that on Mars there are pathogens, organisms which, if transported to the terrestrial environment, might do enormous biological damage.[45]
He makes the analogy of the plot twist in The War of the Worlds, by H.G. Wells, where Earth pathogens made the Martian invaders of Earth sick and then die. Perhaps, he suggests, the same could happen to us on Earth if we return samples containing micro-organisms from Mars. On the one hand, he points out, this possibility seems unlikely because of the lack of contact between the two planets and because pathogens adapt to their host. On the other hand, the lack of contact also means that we would have never evolved any defences against any pathogens.
Pathogens, when they adapt to a host, normally evolve to be less rather than more lethal. Also, some pathogens - such as Legionnaire's disease - attack humans using essentially the same mechanism they use to infect other microbes (in this case, amoebae) so a disease of microbes on Mars could become a pathogen of animals on Earth.
Ledeberg wrote [46]
Whether a microorganism from Mars exists and could attack us is more conjectural. If so, it might be a zoonosis to beat all others. On the one hand, how could microbes from Mars be pathogenic for hosts on Earth when so many subtle adaptations are needed for any new organisms to come into a host and cause disease? On the other hand, microorganisms make little besides proteins and carbohydrates, and the human or other mammalian immune systems typically respond to peptides or carbohydrates produced by invading pathogens. Thus, although the hypothetical parasite from Mars is not adapted to live in a host from Earth, our immune systems are not equipped to cope with totally alien parasites: a conceptual impasse
This possibility has been confirmed in all the later studies, as the worst-case scenario. Other possibilities have also been raised such as micro-organisms that have harmful effects on crops, or that disrupt natural cycles, and pathogens that infect other micro-organisms.[47][48][49][50][51]
As a result, the possibility of new human pathogens, or environmental disruption due to back contamination, is considered to be of extremely low probability but can't yet be ruled out completely.
Later in Cosmos (1980) Carl Sagan wrote:
Perhaps Martian samples can be safely returned to Earth. But I would want to be very sure before considering a returned-sample mission.[52]
The PPO, NASA, and ESA views are similar to this. The findings were that with present-day technology, Martian samples can be safely returned to Earth provided the right precautions are taken.
The risks of environmental disruption resulting from the inadvertent contamination of Earth with putative martian microbes are still considered to be low. But since the risk cannot be demonstrated to be zero, due care and caution must be exercised in handling any martian materials returned to Earth.
Suggested precautions for sample returns
Space agencies have already had experience with returning samples thought to be a back contamination risk during the Apollo era, when samples were returned for the first time by Apollo 11. At the time, it was thought that there was a low probability of life on the Moon. The precautions taken then were inadequate by modern standards, however. The regulations used then have been rescinded, and new regulations would be needed.[54] A different approach would be needed for a modern sample return.
Chain of contact
The sample return mission would be designed to break the chain of contact between Mars and the exterior of the sample container, for instance, by sealing the returned container inside another larger container in the vacuum of space before return to Earth.[47][55] In order to eliminate the risk of parachute failure, the current plan is to return the capsule to the Earth without the use of parachutes: the capsule will fall at terminal velocity and the impact will be cushioned by the capsule's thermal protection system. The sample container would be designed to withstand the force of the impact.[55]
Receiving facility
To receive the returned samples, NASA has proposed to build a biohazard containment facility, known as the Mars Sample Return Receiving facility (MSRRF).[56]
The proposed sample return facility must be a biohazard level 4 laboratory. However, the facility must also contain unknown biohazards, as the sizes of any putative Martian micro-organisms are currently unknown. In consideration of this, the ESF proposed additional requirements. Ideally it should contain particles of 0.01 µm, or larger, and release of a particle 0.05 µm or larger is unacceptable under any circumstances.[57]
The reason for this extremely small size limit of 0.01 µm is because of the consideration of Gene Transfer Agents (GTAs). These randomly incorporate segments of the host genome and can transfer them to other evolutionarily distant hosts, and do that without killing the new host. In this way many archaea and bacteria can swap DNA with each other. This raises the possibility that Martian life, if it has a common origin with Earth life in the distant past, could swap DNA with Earth micro-organisms in the same way.
In one striking experiment first reported in 2010, researchers left GTAs (conferring antibiotic resistance) and marine bacteria overnight in natural conditions and found that by the next day up to 47% of the bacteria had incorporated the genetic material from the GTAs.[58][59]
The reason for the 0.05 µm limit is because of the discovery of ultramicrobacteria as small as 0.2 µm across, and bearing in mind that these smallest size limits may be reduced further in the future..
It also must double as a clean room to preserve the science value of the samples. The problem here is that, while it is relatively easy to simply contain the samples once returned to Earth, researchers would also want to remove parts of the sample and use them in experiments. During all these handling procedures, the samples would need to be contained to prevent contamination of Earth. However at the same time the samples would need to be kept free from contamination by Earth micro-organisms and biological material; even a single amino acid of Earth origin could confuse the analysis. This introduces conflicting requirements. They surely can be reconciled but to date, no facility has had to do this, so new building requirements would need to be imposed.
A clean room is normally kept at a higher pressure than the external environment to keep contaminants out, and a biohazard laboratory is kept at a lower pressure to keep the biohazards in. The challenge is to combine these in a single building. Solutions suggested include a triple walled containment facility, and one of the suggestions includes extensive use of robot handlers for the samples.[60][61][62][63]
Timescale for building the facility
The short timescale of two years used for the quarantine facilities for Apollo 11 is now considered to be too short and probably the main reason why the facilities failed to contain the samples adequately even by the standards of the time, with two failures of containment, one at splashdown and the other in Houston. The facility would be expected to take some years from design to completion.[64][65] Preliminary studies have warned that it may take as many as 7 to 10 years to get it operational and an additional two years is recommended for the staff to become accustomed to the facilities.[47][66]
Dissenting views on back contamination
The International Committee Against Mars Sample Return[67] maintains that it is not possible to return samples to Earth safely at this stage. Their main reason for saying this is the novelty of the containment procedures required combined with the possibility of human error and mission design mistakes, either during the return flight or after return of the samples. They urge more in situ studies on Mars first, and preliminary biohazard testing in space before the samples are returned to Earth.
At the other extreme, Robert Zubrin (Mars surface colonization advocate and director of the Mars Society) maintains that the risk of back contamination has no scientific validity. He supports this using an argument based on the possibility of transfer of life from Earth to Mars on meteorites.[68][69]
For more about these issues see Planetary Protection - Controversies
Legal process of approval for Mars sample return
Margaret Race has examined in detail the legal process of approval for a MSR.[54] She found that under the National Environmental Policy Act (NEPA) (which did not exist in the Apollo era) a formal environment impact statement is likely to be required, and public hearings during which all the issues would be aired openly. This process is likely to take up to several years to complete.
During this process, she found, the full range of worst accident scenarios, impact, and project alternatives would be played out in the public arena. Other agencies such as the Environment Protection Agency, Occupational Health and Safety Administration, etc., may also get involved in the decision making process.
The laws on quarantine will also need to be clarified as the regulations for the Apollo program were rescinded. In the Apollo era, NASA delayed announcement of its quarantine regulations until the day Apollo was launched, so bypassing the requirement for public debate - something that would be unlikely to be tolerated today.
It is also probable that the presidential directive NSC-25 will apply which requires a review of large scale alleged effects on the environment and is carried out subsequent to the other domestic reviews and through a long process, leads eventually to presidential approval of the launch.
Then apart from those domestic legal hurdles, there are numerous international regulations and treaties to be negotiated in the case of a Mars Sample Return, especially those relating to environmental protection and health. She concluded that the public of necessity has a significant role to play in the development of the policies governing Mars Sample Return.
Alternatives to sample returns
Several exobiologists have suggested that a Mars sample return is not necessary at this stage, and that it is better to focus more on in situ studies on the surface first. Although it is not their main motivation, this approach of course also eliminates back contamination risks.
Some of these exobiologists advocate more in situ studies followed by a sample return in the near future. Others go as far as to advocate in situ study instead of a sample return at the present state of understanding of Mars.[70][71][72]
Their reasoning is that life on Mars is likely to be hard to find. Any present day life is likely to be sparse and occur in only a few niche habitats. Past life is likely to be degraded by cosmic radiation over geological time periods if exposed in the top few meters of the Mars surface. Also, only certain special deposits of salts or clays on Mars would have the capability to preserve organics for billions of years. So, they argue, there is a high risk that a Mars sample return at our current stage of understanding would return samples that are no more conclusive about the origins of life on Mars or present day life than the Martian meteorite samples we already have.
Another consideration is the difficulty of keeping the sample completely free from Earth life contamination during the return journey and during handling procedures on Earth. This might make it hard to show conclusively that any biosignatures detected does not result from contamination of the samples.
Instead they advocate sending more sensitive instruments on Mars surface rovers. These could examine many different rocks and soil types, and search for biosignatures on the surface and so examine a wide range of materials which could not all be returned to Earth with current technology at reasonable cost.
A sample return to Earth would then be considered at a later stage, once we have a reasonably thorough understanding of conditions on Mars, and possibly have already detected life there, either modern or past life, though biosignatures and other in situ analysis.
Instruments under development for in situ analysis
- NASA Marshall Space Flight Center is leading a research effort to develop a Miniaturized Variable Pressure Scanning Electron Microscope (MVP-SEM) for future lunar and martian missions.[73]
- Several teams, including Jonathan Rothberg, and J. Craig Venter, are separately developing solutions for sequencing alien DNA directly on the Martian surface itself.[74][75][76][77]
- Levin is working on updated versions of the Labeled release instrument flown on Viking. For instance versions that rely on detecting chirality. This is of special interest because it can enable detection of life even if it is not based on standard life chemistry.[78]
- The Urey Mars Organic and Oxidant Detector instrument for detection of biosignatures has been descoped, but was due to be flown on ExoMars in 2018. It is designed with much higher levels of sensitivity for biosignatures than any previous instruments[70][79][80]
- Astrobionibbler - this is a smaller instrument proposed to replace Urey on ExoMars. It is capable of detecting a single amino acid in a gram of soil.[31]
Study and analyses from orbit
During the “Exploration Telerobotics Symposium" in 2012 experts on telerobotics from industry, NASA and academics met to discuss telerobotics, and its applications to space exploration. Amongst other issues, particular attention was given to Mars missions and a Mars sample return.
They came to the conclusion that telerobotic approaches could permit direct study of the samples on the Mars surface via telepresence from Mars orbit, permitting rapid exploration and use of human cognition to take advantage of chance discoveries and feedback from the results obtained so far.[81]
They found that telepresence exploration of Mars has many advantages. The astronauts have near real-time control of the robots, and can respond immediately to discoveries. It also prevents contamination both ways and has mobility benefits as well.[82]
Return of the sample to orbit has the advantage that it permits analysis of the sample without delay, to detect volatiles that may be lost during a voyage home. This was the conclusion of a meeting of researchers at the NASA Goddard Space Flight Center in 2012. [83]
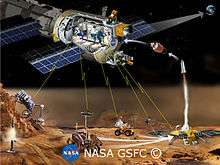
"One possible scenario for surface exploration of Mars via LLT could be the deployment of twin telerobotic rovers on the surface with high-definition visual tools to allow low-latency communication and rapidly adaptable operation from an on-orbit crew for field astrobiology. Such “tele-rovers” could be equipped with instruments for detailed in situ reconnaissance and capabilities for recovering and sending selected samples to the human-tended on-orbit spacecraft for preliminary screening by means of lab analysis by resident astronauts. In the case of samples of biological significance, very rapid encapsulation and recovery of the sample materials at the spacecraft in orbit are required and this is enabled by this approach. Most of the required technology already exists for terrestrial telerobotics exploration of Earth, although the TRL would have to be advanced and validated for operations on Mars"[81]
Similar methods could be used to directly explore other biologically sensitive locations such as Europa, Titan, or Enceladus, once a human presence in the vicinity becomes possible.
See also
References
- ↑ COSPAR Workshop on Planetary Protection for Outer Planet Satellites and Small Solar System Bodies European Space Policy Institute (ESPI), 15–17 April 2009
- ↑ COSPAR power point type presentation, gives good overview of the detailed category decisions
- ↑ Chang, Kenneth (December 9, 2013). "On Mars, an Ancient Lake and Perhaps Life". New York Times.
- ↑ Various (December 9, 2013). "Science - Special Collection - Curiosity Rover on Mars". Science.
- ↑ Experimental evidence for the formation of liquid saline water on Mars , Erik Fischer, Germán M. Martínez, Harvey M. Elliott, Nilton O. Rennó, Geophysical research letters, 7 July 2014 DOI: 10.1002/2014GL060302 "Finally, our results indicate that liquid water could form on the surface during the spring where snow has been deposited on saline soils [Martínez et al., 2012; Möhlmann, 2011]. These results have important implications for the understanding of the habitability of Mars because liquid water is essential for life as we know it, and halophilic terrestrial bacteria can thrive in brines"
- ↑ Water and Brines on Mars: Current Evidence and Implications for MSL G. M. Martínez1 and N. O. Renno, Space Science Reviews, 2013
- ↑ Summons, Roger E.; Amend, Jan P.; Bish, David; Buick, Roger; Cody, George D.; Des Marais, David J.; Dromart, Gilles; Eigenbrode, Jennifer L.; et al. (2011). "Preservation of Martian Organic and Environmental Records: Final Report of the Mars Biosignature Working Group". Astrobiology. 11 (2): 157–81. Bibcode:2011AsBio..11..157S. doi:10.1089/ast.2010.0506. PMID 21417945.
There is general consensus that extant microbial life on Mars would probably exist (if at all) in the subsurface and at low abundance.
- ↑ Didymus, JohnThomas (January 21, 2013). "Scientists find evidence Mars subsurface could hold life". Digital Journal – Science.
There can be no life on the surface of Mars because it is bathed in radiation and it's completely frozen. However, life in the subsurface would be protected from that. - Prof. Parnell.
- ↑ "Mars: 'Strongest evidence' planet may have supported life, scientists say". BBC News. January 20, 2013.
- ↑ Michalski, Joseph R.; Cuadros, Javier; Niles, Paul B.; Parnell, John; Deanne Rogers, A.; Wright, Shawn P. (2013). "Groundwater activity on Mars and implications for a deep biosphere". Nature Geoscience. 6 (2): 133–8. Bibcode:2013NatGe...6..133M. doi:10.1038/ngeo1706.
- ↑ RADIATIVE HABITABLE ZONES IN MARTIAN POLAR ENVIRONMENTS "Finally there are other harmful radiation sources reaching Mars: ionizing and neutron radiation caused by galactic cosmic radiation and solar particle events. Due to the lack of a magnetic field and the low shielding of the Martian atmosphere (the Martian overhead airmass is 16 g cm-2 instead of the terrestrial 1000 g cm-2) the doses of ionizing radiation at the surface of Mars reach values about 100 times higher than those on the Earth. However, since a great variety of microbes tolerate this type of radiation at similar or even greater doses than those found on Mars, ionizing radiation cannot be considered a limiting factor for microbial life on Mars and thus here we will limit our study to solar UV shielding and VIS radiation pentration."
- ↑ Space Station Research Shows That Hardy Little Space Travelers Could Colonize Mars
- ↑ NASA Press Release, May 2014"In another investigation, spores of Bacillus pumilus SAFR-032 and another spore-forming bacteria, Bacillus subtilis 168, were dried on pieces of spacecraft-quality aluminum and subjected for 1.5 years to the vacuum of space, cosmic and extraterrestrial solar radiation and temperature fluctuations on EuTEF. These samples also were subjected to a simulated Martian atmosphere using EuTEF. Most of the organisms exposed to solar UV radiation in space and in the Mars spectrum were killed, but when UV rays were filtered out and samples were kept in the dark, about 50 percent or more of those subjected to other space- and Mars-like conditions survived. That makes it likely that spores could survive a trip on a spacecraft to Mars if they are sheltered against solar radiation, perhaps in a tiny pocket of the spacecraft surface or underneath a layer of other spores."
- ↑ Debus, A. (2005). "Estimation and assessment of Mars contamination". Advances in Space Research. 35 (9): 1648–53. Bibcode:2005AdSpR..35.1648D. doi:10.1016/j.asr.2005.04.084. PMID 16175730.
- ↑ MEPAG Special Regions-Science Analysis Group; Beaty, D.; Buxbaum, K.; Meyer, M.; Barlow, N.; Boynton, W.; Clark, B.; Deming, J.; Doran, P. T.; et al. (2006). "Findings of the Mars Special Regions Science Analysis Group". Astrobiology. 6 (5): 677–732. Bibcode:2006AsBio...6..677M. doi:10.1089/ast.2006.6.677. PMID 17067257.
- ↑ Christopher P. McKay Planetary Ecosynthesis on Mars: Restoration Ecology and Environmental Ethics NASA Ames Research Center
- ↑ Baldwin, Emily (26 April 2012). "Lichen survives harsh Mars environment". Skymania News. Retrieved 27 April 2012.
- ↑ de Vera, J.-P.; Kohler, Ulrich (26 April 2012). "The adaptation potential of extremophiles to Martian surface conditions and its implication for the habitability of Mars" (PDF). European Geosciences Union. Retrieved 27 April 2012.
- ↑ Cassini samples the icy spray of Enceladus' water plumes; June 22, 2011
- ↑ Cassini Tastes Organic Material at Saturn's Geyser Moon; March 12, 2008
- ↑ What Makes Us Think There is an Ocean Beneath Europa's Icy Crust?, Paragraphs 4 - 7
- ↑ Water plumes spark a race to Jupiter moon Europa, Lisa Grossman, New Scientist 31 December 2013
- ↑ Hubble Space Telescope Sees Evidence of Water Vapor Venting off Jupiter Moon, Paragraph 4; December 12, 2013
- ↑ Signs of Europa Plumes Remain Elusive in Search of Cassini Data; December 17, 2014
- ↑ NASA Data Shed New Light About Water and Volcanoes on Mars; November 9, 2010
- ↑ NASA Confirms Evidence That Liquid Water Flows on Today’s Mars, Paragraphs 1 - 5; September 28, 2015
- ↑ The Overprotection of Mars?, astrobio.net, Andrew Williams - Nov 18, 2013 - has links to the papers
- ↑ The Overprotection of Mars?, astrobio.net, Andrew Williams - Nov 18, 2013 - summarizes both papers on the subject, with links to originals
- ↑ Appropriate protection of Mars, Nature, Catherine Conley and John Rummel
- ↑ If There Are Microbes on Mars, We Might Have Put Them There
- 1 2 Michael Schirber Searching for Organics in a Nibble of Soil Astrobiology Magazine, 18th February 2013
- ↑ Isolating "uncultivable" microorganisms in pure culture in a simulated natural environment.
- ↑ Archaeal diversity analysis of spacecraft assembly clean rooms, he ISME Journal (2008) 2, 115–119; doi:10.1038/ismej.2007.98
- 1 2 Rachel Courtland Should Mars be treated like a wildlife preserve? New Scientist, February 2009 - see "Martian rabbit problem" section
- 1 2 Queens University Belfast scientist helps NASA Mars project "No-one has yet proved that there is deep groundwater on Mars, but it is plausible as there is certainly surface ice and atmospheric water vapour, so we wouldn't want to contaminate it and make it unusable by the introduction of micro-organisms."
- ↑ Meltzer, Michael (May 31, 2012). When Biospheres Collide: A History of NASA's Planetary Protection Programs. pp. 46–51. ISBN 978-0-16-085327-2.
- 1 2 When Biospheres Collide - a history of NASA'S Planetary Protection Programs, Michael Meltzer , May 31, 2012, see Chapter 7, Return to Mars - final section: "Should we do away with human missions to sensitive targets"
- ↑ Safe on Mars page 37"Martian biological contamination may occur if astronauts breathe contaminated dust or if they contact material that is introduced into their habitat. If an astronaut becomes contaminated or infected, it is conceivable that he or she could transmit Martian biological entities or even disease to fellow astronauts, or introduce such entities into the biosphere upon returning to Earth. A contaminated vehicle or item of equipment returned to Earth could also be a source of contamination."
- ↑ Mars and the Moon (C. A. Conley & J. D. Rummel Acta Astronautica 63 1025–1030 (2008))
- ↑ Biological contamination studies of lunar landing sites: implications for futureplanetary protection and life detectionon the Moon and Mars, D.P. Glavin, J.P. Dworkin, M. Lupisella, G. Kminek and J.D. Rummel,International Journal of Astrobiology (2004) doi:10.1017/S1473550404001958
- ↑ Almost Being There: Why the Future of Space Exploration Is Not What You Think
- ↑ First Exploration Telereobotics Symposium
- ↑ [HERRO: A Science-Oriented Strategy for Crewed Missions Beyond LEO HERRO: A Science-Oriented Strategy for Crewed Missions Beyond LEO]
- ↑ European Science Foundation - Mars Sample Return backward contamination - strategic advice July, 2012, ISBN 978-2-918428-67-1 - see 2. From remote exploration to returning samples. (for more details of the document see abstract )
- ↑ Carl Sagan,The Cosmic Connection - an Extraterrestrial Perspective (1973) ISBN 0521783038
- ↑ Parasites Face a Perpetual Dilemma
- 1 2 3 European Science Foundation - Mars Sample Return backward contamination - Strategic advice and requirements July, 2012, ISBN 978-2-918428-67-1. (for more details of the document see abstract )
- ↑ Joshua Lederberg Parasites Face a Perpetual Dilemma Volume 65, Number 2, 1999 / American Society for Microbiology News 77.
- ↑ Assessment of Planetary Protection Requirements for Mars Sample Return Missions (Report). National Research Council. 2009.
- ↑ http://mepag.nasa.gov/reports/iMARS_FinalReport.pdf Preliminary Planning for an International Mars Sample Return Mission Report of the International Mars Architecture for the Return of Samples (iMARS) Working Group June 1, 2008
- ↑ http://planetaryprotection.nasa.gov/summary/msr Mars Sample Return: Issues and Recommendations. Task Group on Issues in Sample Return. National Academies Press, Washington, DC (1997).
- ↑ Carl Sagan Cosmos Random House Publishing Group, 6 Jul 2011
- ↑ Assessment of Planetary Protection Requirements for Mars Sample Return Missions (Report). National Research Council. 2009.
- 1 2 M. S. Race Planetary Protection, Legal Ambiguity, and the Decision Making Process for Mars Sample Return Adv. Space Res. vol 18 no 1/2 pp (1/2)345-(1/2)350 1996
- 1 2 http://mepag.jpl.nasa.gov/meeting/mar-10/Li2-MSR_Dis-for-MEPAG3-17_tech_updates.pdf Mars Sample Return Discussions As presented on February 23, 2010
- ↑ Mars Sample Return Receiving Facility
- ↑ European Science Foundation - Mars Sample Return backward contamination - Strategic advice and requirements
- ↑ Amy Maxmen Virus-like particles speed bacterial evolution published online 30 September 2010
- ↑ Lauren D. McDaniel, Elizabeth Young, Jennifer Delaney, Fabian Ruhnau, Kim B. Ritchie, John H. Paul High Frequency of Horizontal Gene Transfer in the Oceans Science 1 October 2010: Vol. 330 no. 6000 p. 50 doi:10.1126/science.1192243
- ↑ Mars Sample Return Receiving Facility - A Draft Test Protocol for Detecting Possible Biohazards in Martian Samples Returned to Earth (PDF) (Report). 2002.
A Sample Return Facility will require combining technologies used for constructing maximum containment laboratories (e.g. Biosafety Level 4 labs), which will be needed to ensure protection of Earth from the Mars samples, with cleanroom technologies, which will be needed to protect the Mars samples from Earth contamination.
• Such an integrated facility is not currently available.
Planetary Protection Requires Negative Air Flow to Protect Against Environmental Contamination Planetary Science and Planetary Protection Require Positive Air Flow to Protect Samples from Terrestrial Contamination - ↑ A Draft Test Protocol for Detecting Possible Biohazards in Martian Samples Returned to Earth
- ↑ CLEANROOM ROBOTICS – APPROPRIATE TECHNOLOGY FOR A SAMPLE RECEIVING FACILITY ? 2005 update on the Draft Test Protocol .
- ↑ 2010 Mars Sample Return Orbiter decadal survey:
The NASA Planetary Protection Officer commissioned the development of a draft test protocol that would represent one "necessary and sufficient" approach to evaluate the safety of the samples while safeguarding the purity of the samples from terrestrial contamination. A Draft Test Protocol for Detecting Possible Biohazards in Martian Samples Returned to Earth was published in October 2002 [7]. In 2003, three architectural design teams independently examined the scope, approach, cost, and technology required for the SRF, using the Draft Test Protocol for requirements. The approaches varied from allrobotic handling of samples to more traditional glove box implementations. The studies indicated that the principles and techniques required are generally mature. Biosafety laboratories, the NASA Lunar Sample Facility, pharmaceutical laboratories, and electronic fabrication cleanrooms perform most of the required individual functions. However, there are some areas needing early development, such as ensuring sample preservation and bio-safety together, representing new challenges that were addressed by techniques like dual-walled containers (and gloves) with positive pressure clean inert gas in between the walls. This, as well as some further development in ultra-clean sample manipulation, safe and pure transport of samples, and sample sterilization techniques, are planned in the technology program
- ↑ "7: "Sample-Receiving Facility and Program Oversight"". Assessment of Planetary Protection Requirements for Mars Sample Return Missions (Report). National Research Council. 2009. p. 59.
It has been estimated that the planning, design, site selection, environmental reviews, approvals, construction, commissioning, and pre-testing of a proposed safe room facility (SRF) will occur 7 to 10 years before actual operations begin. In addition, 5 to 6 years will likely be required for refinement and maturation of SRF-associated technologies for safely containing and handling samples to avoid contamination and to further develop and refine biohazard-test protocols. Many of the capabilities and technologies will either be entirely new or will be required to meet the unusual challenges of integration into an overall (end-to-end) Mars sample return program.
- ↑ Mars Sample Return: Issues and Recommendations (Planetary Protection Office Summary) Task Group on Issues in Sample Return. National Academies Press, Washington, DC (1997)
- ↑ "7: "Sample-Receiving Facility and Program Oversight"". Assessment of Planetary Protection Requirements for Mars Sample Return Missions (Report). National Research Council. 2009. p. 59.
It has been estimated that the planning, design, site selection, environmental reviews, approvals, construction, commissioning, and pre-testing of a proposed SRF will occur 7 to 10 years before actual operations begin.17,18,19 In addition, 5 to 6 years will likely be required for refinement and maturation of SRF-associated technologies for safely containing and handling samples to avoid contamination and to further develop and refine biohazard-test protocols. Many of the capabilities and technologies will either be entirely new or will be required to meet the unusual challenges of integration into an overall (end-to-end) Mars sample return program.
- ↑ International Committee Against Mars Sample Return
- ↑ Robert Zubrin "Contamination From Mars: No Threat", The Planetary Report July/Aug. 2000, P.4–5
- ↑ transcription of a tele-conference interview with ROBERT ZUBRIN conducted on March 30, 2001 by the class members of STS497 I, "Space Colonization"; Instructor: Dr. Chris Churchill
- 1 2 Jeffrey L. Bada, Andrew D. Aubrey, Frank J. Grunthaner, Michael Hecht, Richard Quinn, Richard Mathies, Aaron Zent, John H. Chalmers Seeking signs of life on mars: in situ investigations as prerequisites to sample return missions Independent Contribution to the Mars Decadal Survey Panel
- ↑ Mars Exploration Strategies: Forget About Sample Return D. A. Paige, Dept. of Earth and Space Sciences, UCLA, Los Angeles, CA 90095
- ↑ Future Mars Missions: Can Humans Trump Robots?
- ↑ Gaskin, J.A.; Jerman, G.; Gregory, D.; Sampson, A.R., Miniature Variable Pressure Scanning Electron Microscope for in-situ imaging & chemical analysis Aerospace Conference, 2012 IEEE , vol., no., pp.1,10, 3–10 March 2012 doi: 10.1109/AERO.2012.6187064
- ↑ Mars Sample Return Mission? Naaah… Just Beam Back Martian DNA
- ↑ Biomedicine News Genome Hunters Go After Martian DNA
- ↑ Researchers Design a DNA Sequencing Microchip for Detecting Life on Mars Science Tech Daily, July 9, 2013
- ↑ Radiation Resistance of Sequencing Chips for in situ Life Detection Christopher E. Carr, Holli Rowedder, Clarissa S. Lui, Ilya Zlatkovsky, Chris W. Papalias, Jarie Bolander, Jason W. Myers, James Bustillo, Jonathan M. Rothberg, Maria T. Zuber, and Gary Ruvkun. Astrobiology. June 2013, 13(6) 560-569. doi:10.1089/ast.2012.0923
- ↑ A. D. Anbar1 and G. V. Levin A CHIRAL LABELED RELEASE INSTRUMENT FOR IN SITU DETECTION OF EXTANT LIFE., Concepts and Approaches for Mars Exploration (2012)
- ↑ Andrew D. Aubrey,1 John H. Chalmers, Jeffrey L. Bada, Frank J. Grunthaner, Xenia Amashukeli, Peter Willis, Alison M. Skelley, Richard A. Mathies, Richard C. Quinn, Aaron P. Zent, Pascale Ehrenfreund, Ron Amundson, Daniel P. Glavin, Oliver Botta, Laurence Barron,1 Diana L. Blaney, Benton C. Clark,11 Max Coleman, Beda A. Hofmann,12 Jean-Luc Josset,1 Petra Rettberg, Sally Ride, François Robert, Mark A. Sephton, and Albert Yen1 The Urey Instrument: An Advanced In Situ Organic and Oxidant Detector for Mars Exploration ASTROBIOLOGY Volume 8, Number 3, 2008
- ↑ J.L. Bada ·P. Ehrenfreund ·F. Grunthaner ·D. Blaney ·M. Coleman · A. Farrington ·A. Yen ·R. Mathies·R. Amudson ·R. Quinn ·A. Zent·S. Ride · L. Barron ·O. Botta ·B. Clark ·D. Glavin ·B. Hofmann · J.L. Josset·P. Rettberg · F. Robert ·M. Sephton Urey: Mars Organic and Oxidant Detector Space Sci Rev (2008) 135: 269–279
- 1 2 LOW-LATENCY TELEROBOTICS FROM MARS ORBIT: THE CASE FOR SYNERGY BETWEEN SCIENCE AND HUMAN EXPLORATION, Concepts and Approaches for Mars Exploration (2012)
- ↑ Space Exploration Enabled by Telepresence: Combining Science and Human Exploration Based on Findings from: "Exploration Telerobotics Symposium" May 2-3, 2012 NASA Goddard Space Flight Center
- ↑ Space Exploration Via Telepresence: The Case for Synergy Between Science and Human Exploration, Findings and Observations from: "Exploration Telerobotics Symposium" May 2-3, 2012 NASA Goddard Space Flight Center