Organic peroxide
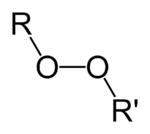
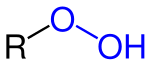

Organic peroxides are organic compounds containing the peroxide functional group (ROOR′). If the R′ is hydrogen, the compounds are called organic hydroperoxides. Peresters have general structure RC(O)OOR. The O−O bond easily breaks, producing free radicals of the form RO•. Thus, organic peroxides are useful as initiators for some types of polymerisation, such as the epoxy resins used in glass-reinforced plastics. MEKP and benzoyl peroxide are commonly used for this purpose. However, the same property also means that organic peroxides can either intentionally or unintentionally initiate explosive polymerisation in materials with unsaturated chemical bonds, and this process has been used in explosives. Organic peroxides, like their inorganic counterparts, are powerful bleaching agents.[1]

Properties
The O−O bond length in peroxides is about 1.45 Å, and the R−O−O angles (R = H, C) are about 110° (water-like). Characteristically, the C−O−O−R (R = H, C) dihedral angles are about 120°. The O−O bond is relatively weak, with a bond dissociation energy of 45–50 kcal/mol (190–210 kJ/mol), less than half the strengths of C−C, C−H, and C−O bonds.[2]
The oxidizing tendency of peroxides is related to the electronegativity of the substituents. Electrophilic peroxides are stronger oxygen-atom transfer agents. For hydroperoxides, the oxygen-atom donor tendency correlates with the acidity of the O−H bond. Thus, the order of oxidizing power is CF3CO3H > CH3CO3H > H2O2.
Active oxygen in peroxides
Each peroxy group is considered to contain one active oxygen atom. The concept of active oxygen content is useful for comparing the relative concentration of peroxy groups in formulations, which is related to the energy content. In general, energy content increases with active oxygen content, and thus the higher the molecular weight of the organic groups, the lower the energy content and, usually, the lower the hazard.
The term active oxygen is used to specify the amount of peroxide present in any organic peroxide formulation. One of the oxygen atoms in each peroxide group is considered "active". The theoretical amount of active oxygen can be described by the following equation:[3]
- A[O]theoretical (%) = 16p/m × 100,
where p is the number of peroxide groups in the molecule, and m is the molecular mass of the pure peroxide.
Organic peroxides are often sold as formulations that include one or more phlegmatizing agents. That is, for safety sake or performance benefits the properties of an organic peroxide formulation are commonly modified by the use of additives to phlegmatize (desensitize), stabilize, or otherwise enhance the organic peroxide for commercial use. Commercial formulations occasionally consist of mixtures of organic peroxides, which may or may not be phlegmatized.
Organic peroxides may be in the solid or liquid state. There are no organic peroxides in the vapor state. Occasionally liquid organic peroxides are desensitized by mixing with solid materials so that the mixture behaves as if it is a solid.
Thermal decomposition of organic peroxides
Unlike most other chemicals, the purpose of a peroxide is to decompose. In doing so it generates useful radicals that can initiate polymerization to make polymers, modify polymers by grafting or visbreaking, or cross-link polymers to create a thermoset. When used for these purposes, the peroxide is highly diluted, so the heat generated by the exothermic decomposition is safely absorbed by the surrounding medium (e.g. polymer compound or emulsion). But when a peroxide is in a more pure form, the heat evolved by its decomposition may not dissipate as quickly as it is generated, which can result in increasing temperature, which further intensifies the rate of exothermic decomposition. This can create a dangerous situation known as a self-accelerating decomposition.
A self-accelerating decomposition occurs when the rate of peroxide decomposition is sufficient to generate heat at a faster rate than it can be dissipated to the environment. Temperature is the main factor in the rate of decomposition. The lowest temperature at which a packaged organic peroxide will undergo a self-accelerating decomposition within a week is defined as the self-accelerating decomposition temperature (SADT).
Industrial uses
In polymer chemistry
Organic peroxides find numerous applications, often involving similar chemistry. Thus, peroxides serve as accelerators, activators, cross-linking agents, curing and vulcanization agents, hardeners, polymerisation initiators, and promoters. Drying oils, as found in many paints and varnishes function via the formation of hydroperoxides.
Methyl ethyl ketone peroxide, benzoyl peroxide and to a smaller degree acetone peroxide are used as initiators for radical polymerisation of some resins, e.g. polyester and silicone, often encountered when making fiberglass.
Bleaching and disinfecting agents
Benzoyl peroxide and hydrogen peroxide are used as bleaching and "maturing" agents for treating flour to make its grain release gluten more easily; the alternative is letting the flour slowly oxidize by air, which is too slow for the industrialized era. Benzoyl peroxide is an effective topical medication for treating most forms of acne.
In the synthesis of organic compounds
Many organic compounds are prepared using peroxides, most famously epoxides from alkenes. Tert-butyl hydroperoxide (TBHP) is an organic-soluble oxidant employed in a variety of metal-catalyzed oxidations, such as the Halcon process (to give propylene oxide) and the Sharpless epoxidation.
Explosives
Paramilitaries use Acetone peroxide in explosives, despite its instability, because of its ease of manufacture and lack of telltale nitrate signature. It is notorious for susceptibility to heat, friction, and shock.[4] Hexamethylene triperoxide diamine (HMTD) and Methyl ethyl ketone peroxide (MEKP) are other widely known explosive organic peroxides.
Preparation
By autoxidation
Most peroxides are generated by the addition of O2 to hydrocarbons. Compounds with allylic and benzylic C−H bonds are amenable to this method.[5] Cumene hydroperoxide is an intermediate in the cumene process of industrial synthesis of phenol. Ethers are susceptible to this reaction, a typical example being the formation of diethyl ether peroxide. The photooxidation of dienes affords dialkyl peroxides.
Many organo-metallic compounds insert O2 into the M−C bond. Organolithium and Grignard reagents react with O2 to give hydroperoxides upon hydrolysis. Oxymercuration of alkenes followed by reaction with a hydroperoxide proceeds similarly.
From H2O2
Carboxylic acids react with hydrogen peroxide to give peroxy acids.
Reactions
Some peroxide reactions are:
- Organic reduction to alcohols with lithium aluminium hydride or phosphite esters
- Cleavage to ketones and alcohols in the base catalyzed Kornblum–DeLaMare rearrangement
Safety
As they combine unstably bound oxygen together with hydrogen and carbon in the same molecule, organic peroxides ignite easily and burn rapidly and intensely. The same applies to organic materials contaminated with organic peroxides. Since peroxides can form spontaneously in some materials, some caution must be exercised with such "peroxide forming materials", such as the common ethers diethyl ether, tetrahydrofuran or ethylene glycol dimethyl ether. Acetone peroxide, a powerful explosive, is an unwanted and dangerous byproduct of several chemical reactions, ranging from synthesis of MDMA (where it is a by-product of isosafrole oxidation in acetone) to industrial production of phenol (where the second product of the cumene process, acetone, is partially oxidized to peroxide on the second reaction step). Accidental preparation of organic peroxides can occur by mixing ketone solvents (most commonly acetone) with waste materials containing hydrogen peroxide or other oxidizers and leaving the mixture standing for several hours.
For safe handling of concentrated organic peroxides, an important parameter is temperature of the sample, which should be maintained below the self accelerating decomposition temperature of the compound.[6]
The shipping of organic peroxides is restricted. The US Department of Transportation lists organic peroxide shipping restrictions and forbidden materials in 49 CFR 172.101 Hazardous Materials Table based on the concentration and physical state of the material:
Chemical name | CAS Number | Prohibitions |
---|---|---|
Acetyl acetone peroxide | 37187-22-7 | > 9% by mass active oxygen |
Acetyl benzoyl peroxide | 644-31-5 | solid, or > 40% in solution |
Ascaridole | 512-85-6 | (organic peroxide) |
tert-Butyl hydroperoxide | 75-91-2 | > 90% in solution (aqueous) |
Di-(1-naphthoyl)peroxide | 29903-04-6 | |
Diacetyl peroxide | 110-22-5 | solid, or > 25% in solution |
Ethyl hydroperoxide | 3031-74-1 | |
Iodoxy compounds | dry | |
Methyl ethyl ketone peroxide | 1338-23-4 | > 9% by mass active oxygen in solution |
Methyl isobutyl ketone peroxide | 37206-20-5 | > 9% by mass active oxygen in solution |
See also
External links
- OSH Answers – organic peroxides
- "The Perils of Peroxides". carolina.com. Burlington, NC: Carolina Biological Supply Company. Archived from the original on 2007-12-18.
- Peroxide disposal
- Organic Peroxide Producers Safety Division. Oct. 2011. The Society of the Plastics Industry. 24 Oct. 2011.
References
- ↑ Klenk, Herbert; Götz, Peter H.; Siegmeier, Rainer; Mayr, Wilfried (2005), "Peroxy Compounds, Organic", Ullmann's Encyclopedia of Industrial Chemistry, Weinheim: Wiley-VCH
- ↑ Bach, Robert D.; Ayala, Philippe Y.; Schlegel, H. B. (1996). "A Reassessment of the Bond Dissociation Energies of Peroxides. An ab Initio Study". J. Am. Chem. Soc. 118: 12758–12765. doi:10.1021/ja961838i.
- ↑ "ASTM E298, Standard Test Methods for Assay of Organic Peroxides". ASTM. 2010.
- ↑ See for example the 2006 transatlantic aircraft plot.
- ↑ Knight, H. B.; Swern, Daniel (1963). "Tetralin hydroperoxide". Org. Synth.; Coll. Vol., 4, p. 895.
- ↑ Organic Peroxide Producers Safety Division (2012-08-06). "Safety and Handling of Organic Peroxides" (PDF). The Society of the Plastics Industry, Inc.