Precision agriculture


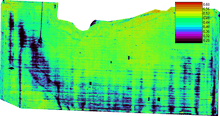
Precision agriculture (PA) or satellite farming or site specific crop management (SSCM) is a farming management concept based on observing, measuring and responding to inter and intra-field variability in crops. The goal of precision agriculture research is to define a decision support system (DSS) for whole farm management with the goal of optimizing returns on inputs while preserving resources.[2][3]
Among these many approaches is a phytogeomorphological approach which ties multi-year crop growth stability/characteristics to topological terrain attributes. The interest in the phytogeomorphological approach stems from the fact that the geomorphology component typically dictates the hydrology of the farm field.[4][5]
The practice of precision agriculture has been enabled by the advent of GPS and GNSS. The farmer's and/or researcher's ability to locate their precise position in a field allows for the creation of maps of the spatial variability of as many variables as can be measured (e.g. crop yield, terrain features/topography, organic matter content, moisture levels, nitrogen levels, pH, EC, Mg, K, etc.).
Precision agriculture has also been enabled by technologies including crop yield monitors mounted on GPS equipped combine harvesters, the development of variable rate technology (VRT) like seeders, sprayers, etc., the development of an array of real-time vehicle mountable sensors that measure everything from chlorophyll levels to plant water status, multi- and hyper-spectral aerial and satellite imagery, from which products like NDVI maps can be made.
Overview
Precision agriculture aims to optimize field-level management with regard to:
- crop science: by matching farming practices more closely to crop needs (e.g. fertilizer inputs);
- environmental protection: by reducing environmental risks and footprint of farming (e.g. limiting leaching of nitrogen);
- economics: by boosting competitiveness through more efficient practices (e.g. improved management of fertilizer usage and other inputs).
Precision agriculture also provides farmers with a wealth of information to:
- build up a record of their farm;
- improve decision-making;
- foster greater traceability
- enhance marketing of farm products
- improve lease arrangements and relationship with landlords
- enhance the inherent quality of farm products (e.g. protein level in bread-flour wheat)
Prescriptive planting
Prescriptive planting is a type of farming system that delivers data-driven planting advice that can determine variable planting rates to accommodate varying conditions across a single field, in order to maximize yield. It has been described as "Big Data on the farm." Monsanto, DuPont and others are launching this technology in the US.[6][7]
Tools
Precision agriculture is usually done as a four-stage process to observe spatial variability:
Data Collection
Geolocating a field enables the farmer to overlay information gathered from analysis of soils and residual nitrogen, and information on previous crops and soil resistivity. Geolocation is done in two ways:
- The field is delineated using an in-vehicle GPS receiver as the farmer drives a tractor around the field.
- The field is delineated on a basemap derived from aerial or satellite imagery. The base images must have the right level of resolution and geometric quality to ensure that geolocation is sufficiently accurate.
Variables
Intra and inter-field variability may result from a number of factors. These include climatic conditions (hail, drought, rain, etc. ), soils (texture, depth, nitrogen levels), cropping practices (no-till farming), weeds and disease. Permanent indicators—chiefly soil indicators—provide farmers with information about the main environmental constants. Point indicators allow them to track a crop’s status, i.e., to see whether diseases are developing, if the crop is suffering from water stress, nitrogen stress, or lodging, whether it has been damaged by ice and so on. This information may come from weather stations and other sensors (soil electrical resistivity, detection with the naked eye, satellite imagery, etc.). Soil resistivity measurements combined with soil analysis make it possible to measure moisture content. Soil resistivity is also a relatively simple and cheap measurement.[8]
Strategies

Using soil maps, farmers can pursue two strategies to adjust field inputs:
- Predictive approach: based on analysis of static indicators (soil, resistivity, field history, etc.) during the crop cycle.
- Control approach: information from static indicators is regularly updated during the crop cycle by:
- sampling: weighing biomass, measuring leaf chlorophyll content, weighing fruit, etc.
- remote sensing: measuring parameters like temperature (air/soil), humidity (air/soil/leaf), wind or stem diameter is possible thanks to Wireless Sensor Networks[9]
- proxy-detection: in-vehicle sensors measure leaf status; this requires the farmer to drive around the entire field.
- aerial or satellite remote sensing: multispectral imagery is acquired and processed to derive maps of crop biophysical parameters. Airbourne instruments are able to measure the amount of plant cover and to distinguish between crops and weeds.[10]
Decisions may be based on decision-support models (crop simulation models and recommendation models), but in the final analysis it is up to the farmer to decide in terms of business value and impacts on the environment.
Implementing practices
New information and communication technologies (NICT) make field-level crop management more operational and easier to achieve for farmers. Application of crop management decisions calls for agricultural equipment that supports variable-rate technology (VRT), for example varying seed density along with variable-rate application (VRA) of nitrogen and phytosanitary products.[11]
Precision agriculture uses technology on agricultural equipment (e.g. tractors, sprayers, harvesters, etc.):
- positioning system (e.g. GPS receivers that use satellite signals to precisely determine a position on the globe);
- geographic information systems (GIS), i.e., software that makes sense of all the available data;
- variable-rate farming equipment (seeder, spreader).
The use of automated or robotic data gathering techniques is on the rise. ROS Agriculture - Software for agricultural robotics.[12]
Usage around the world
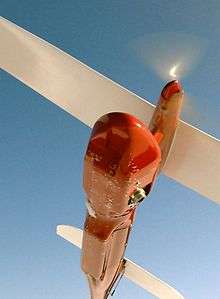
The concept of precision agriculture first emerged in the United States in the early 1980s. In 1985, researchers at the University of Minnesota varied lime inputs in crop fields. It was also at this time that the practice of grid sampling appeared (applying a fixed grid of one sample per hectare). Towards the end of the 1980s, this technique was used to derive the first input recommendation maps for fertilizers and pH corrections. The use of yield sensors developed from new technologies, combined with the advent of GPS receivers, has been gaining ground ever since. Today, such systems cover several million hectares.
In the American Midwest (US), it is associated not with sustainable agriculture but with mainstream farmers who are trying to maximize profits by spending money only in areas that require fertilizer. This practice allows the farmer to vary the rate of fertilizer across the field according to the need identified by GPS guided Grid or Zone Sampling. Fertilizer that would have been spread in areas that don't need it can be placed in areas that do, thereby optimizing its use.
Around the world, precision agriculture developed at a varying pace. Precursor nations were the United States, Canada and Australia. In Europe, the United Kingdom was the first to go down this path, followed closely by France, where it first appeared in 1997-1998. In Latin America the leading country is Argentina, where it was introduced in the middle 1990s with the support of the National Agricultural Technology Institute. The actual scenario of agriculture in Brazil walks towards efficient production with environment protection therefore Embrapa established the Brazilian Precision Agriculture Research Network, with the objective of knowledge generation, tools and technologies development on precision agriculture to soybean, maize, wheat, rice, cotton, pasture, eucalyptus, pines, grapes, peach, orange and sugar cane crops. The development of GPS and variable-rate spreading techniques helped to anchor precision farming[13] management practices. Today, less than 10% of France’s farmers are equipped with variable-rate systems. Uptake of GPS is more widespread, but this hasn’t stopped them using precision agriculture services, which supplies field-level recommendation maps.[14]
Economic and environmental impacts
Precision agriculture, as the name implies, means application of precise and correct amount of inputs like water, fertilizer, pesticides etc. at the correct time to the crop for increasing its productivity and maximizing its yields. Precision agriculture management practices can significantly reduce the amount of nutrient and other crop inputs used while boosting yields. Farmers thus obtain a return on their investment by saving on phytosanitary and fertilizer costs. The second, larger-scale benefit of targeting inputs—in spatial, temporal and quantitative terms—concerns environmental impacts. Applying the right amount of inputs in the right place and at the right time benefits crops, soils and groundwater, and thus the entire crop cycle. Consequently, precision agriculture has become a cornerstone of sustainable agriculture, since it respects crops, soils and farmers. Sustainable agriculture seeks to assure a continued supply of food within the ecological, economic and social limits required to sustain production in the long term. Precision agriculture therefore seeks to use high-tech systems in pursuit of this goal.
A recent article has tried to show that precision agriculture can help farmers in developing countries like India.[15]
Conferences
- InfoAg Conference
- European conference on Precision Agriculture (ECPA) (biennial)
- International Conference on Precision Agriculture (ICPA) (biennial)
See also
- Satellite crop monitoring
- Geostatistics
- Integrated farming
- Precision livestock farming
- Integrated Pest Management
- Nutrient budgeting
- Nutrient management
- Precision viticulture
- Landsat program
- SPOT (satellites)
- PreAgri.com - is an online service for spatial data collection and analysis of agricultural enterprises
Notes
- ↑ "Precision Farming : Image of the Day". earthobservatory.nasa.gov. Retrieved 2009-10-12.
- ↑ McBratney, A., Whelan, B., Ancev, T., 2005. Future Directions of Precision Agriculture. Precision Agriculture, 6, 7-23.
- ↑ Whelan, B.M., McBratney, A.B., 2003. Definition and Interpretation of potential management zones in Australia, In: Proceedings of the 11th Australian Agronomy Conference, Geelong, Victoria, Feb. 2-6 2003.
- ↑ Howard, J.A., Mitchell, C.W., 1985. Phytogeomorphology. Wiley.
- ↑ Kaspar, T.C, Colvin, T.S., Jaynes, B., Karlen, D.L., James, D.E, Meek, D.W., 2003. Relationship between six years of corn yields and terrain attributes. Precision Agriculture, 4, 87-101.
- ↑ Bunge, Jacob (25 February 2014). "Big Data Comes to the Farm, Sowing Mistrust". Wall Street Journal. Retrieved 10 February 2015.
- ↑ "Digital disruption on the farm". The Economist. 24 May 2014. Retrieved 10 February 2015.
- ↑ "Precision Farming Tools: Soil Electrical Conductivity" (PDF). Retrieved Jun 12, 2016.
- ↑ "An example of Wireless Sensor Network node applied to agriculture"
- ↑ "The future of agriculture". The Economist. Retrieved 2016-06-12.
- ↑ Herring, David. "Precision Farming : Feature Articles". earthobservatory.nasa.gov. Retrieved 2009-10-12.
- ↑ Droter, Matt. "Software for agricultural robots.". ROS.org. Retrieved 2016-09-27.
- ↑ "Simon Blackmore: Farming with robots". SPIE Newsroom. Retrieved 2 June 2016.
- ↑ precision agriculture with satellite imagery
- ↑ Anil K. Rajvanshi:"Is precision agriculture the solution to India's farming crisis"
External links
Media related to Precision farming at Wikimedia Commons