Redfield ratio
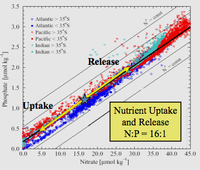
Redfield ratio or Redfield stoichiometry is the atomic ratio of carbon, nitrogen and phosphorus found in phytoplankton and throughout the deep oceans. This empirically developed stoichiometric ratio is found to be C:N:P = 106:16:1. This term is named after the American oceanographer Alfred C. Redfield, who first described this ratio in an article written in 1934 (Redfield 1934). As a Harvard physiologist, Redfield participated in several voyages on board the research vessel Atlantis. Alfred Redfield analyzed thousands of samples of marine biomass across all of the ocean regions. From this research he found that globally the elemental composition of marine organic matter (dead and living) was remarkably constant across all of the regions. The stoichiometric ratios of carbon, nitrogen, phosphorus remain relatively consistent from both the coastal to open ocean regions.
History
For his 1934 paper, Alfred Redfield analyzed nitrate and phosphate data for Atlantic, Indian, Pacific oceans and Barents Sea, including data published by other researchers. In addition, Redfield analyzed data for C, N, and P content in marine plankton, including data collected by other researchers as early 1898.
Redfield’s analysis of the empirical data led to him to a startling discovery: across and within the three oceans and Barents Sea, seawater had the N:P atomic ratio near 20:1 (later corrected to 16:1), and was very similar to the average N:P in plankton. Redfield seemed to be deeply puzzled that “the definite correlation exists between the quantity of nitrate and phosphate occurring in any sample” and thought that “it is pertinent to inquire how these proportions agree with those actually found in various members of the plankton community.” (Redfield 1934)
Redfield foresaw that “relation between the concentration of the various organic derivatives in sea water and the chemical composition of plankton would provide a valuable tool in the analysis of many oceanographic problems.” (Redfield 1934)
Understanding that the problem is akin to the classical the chicken or the egg causality dilemma, Redfield proposed two mutually non-exclusive mechanisms:
I) The N:P in plankton tends to the N:P composition of seawater. Specifically, phytoplankton species with different N and P requirements compete and, as the result, balance each other so that “the ratio of the elements in the plankton as a whole might come to reflect the ratio of the nutrient substances in sea water rather closely” (Redfield 1934).
II) The N:P in seawater “must tend to approach that characteristic of protoplasm in general” (Redfield 1934). Furthermore, Redfield proposed thermostat like scenario in which the activities of nitrogen fixers and denitrifies keep the nitrate to phosphate ratio in the seawater near the requirements in the protoplasm. Considering that at the time little was known about the composition of “protoplasm,” Redfield did not attempt to explain why its N:P ratio should be approximately 16:1.
In 1958, almost a quarter century after first discovering the ratios, Redfield leaned toward the latter mechanism proposing in his seminal manuscript the idea of "the biological control of chemical factors" in the ocean (Redfield, 1958). Redfield proposed that the ratio of Nitrogen to Phosphorus in plankton resulted in the global ocean having a remarkably similar ratio of dissolved nitrate to phosphate (16:1). He considered how the cycles of not just N and P but also C and O could interact to result in this match.
Uses
The research that resulted in this ratio has become a fundamental feature in the understanding of the biogeochemical cycles of the oceans, and one of the key tenets of biogeochemistry. The Redfield ratio is instrumental in estimating carbon and nutrient fluxes in global circulation models. They also help in determining which nutrients are limiting in a localized system, if there is a limiting nutrient. The ratio can also be used to understand the formation of phytoplankton blooms and subsequently hypoxia by comparing the ratio between different regions, such as a comparison of the Redfield Ratio of the Mississippi River to the ratio of the northern Gulf of Mexico.
Explanation
Redfield discovered the remarkable congruence between the chemistry of the deep ocean and the chemistry of living things such as phytoplankton in the surface ocean. Both have N:P ratios of about 16:1 in terms of atoms. When nutrients are not limiting, the molar elemental ratio C:N:P in most phytoplankton is 106:16:1. Redfield thought it wasn't purely coincidental that the vast oceans would have a chemistry perfectly suited to the requirements of living organisms.
In the ocean a large portion of the biomass is found to be nitrogen-rich plankton. Many of these plankton are consumed by other plankton biomass which have similar chemical compositions. This results in a similar nitrogen to phosphorus ratio, on average, for all the plankton throughout the world’s ocean, empirically found to be averaging approximately 16:1. When these organisms sink into the ocean interior, their energy-rich bodies are consumed by bacteria that, in aerobic conditions, oxidize the organic matter to form dissolved inorganic nutrients, mainly carbon dioxide, nitrate, and phosphate.
That the nitrate to phosphate ratio in the interior of all of the major ocean basins is highly similar is possibly due to the residence times of these elements in the ocean relative to the oceans circulation time, roughly 100 000 years for phosphorus and 2000 years for nitrogen.[1] The fact that the residence times of these elements are greater than the mixing times of the oceans (~ 1000 years) [2] can result in the ratio of nitrate to phosphate in the ocean interior remaining fairly constant.
While such arguments can potentially explain why the ratios are fairly constant, they do not address the question why the N:P ratio is nearly 16 and not some other number.
Deviations from the canonical Redfield Ratio
The Redfield ratio was initially derived empirically from measurements of the elemental composition of plankton in addition to the nitrate and phosphate content of seawater collected from a few stations in the Atlantic Ocean. This was later supported by hundreds of independent measurements. However, looking at the composition of individual species of phytoplankton grown under nitrogen or phosphorus limitation shows that this nitrogen to phosphorus ratio can vary anywhere from 6:1 to 60:1. While understanding this problem, Redfield never attempted to explain it with the exception of noting that the N:P ratio of inorganic nutrients in the ocean interior was an average with small scale variability to be expected.
Although the Redfield ratio is remarkably stable in the deep ocean, phytoplankton may have large variations in the C:N:P composition, and their life strategy play a role in the C:N:P ratio, which has made some researchers speculate that the Redfield ratio perhaps is a general average rather than specific requirement for phytoplankton growth (e.g., Arrigo 2005). However, the Redfield ratio was recently found to be related to a homeostatic protein-to-rRNA ratio fundamentally present in both prokaryotes and eukaryotes (Loladze and Elser 2011). Furthermore, the Redfield ratio has been shown to vary at different spatial scales as well as average slightly higher (166:20:1) than Redfield's original estimate (Sterner et al. 2008).
Despite reports that the elemental composition of organisms such as marine phytoplankton in an oceanic region do not conform to the canonical Redfield ratio, the fundamental concept of this ratio continues to remain valid and useful.
Extended Redfield ratio
Some feel that there are other elements, such as potassium, sulfur, zinc, copper, and iron are also important in the ocean chemistry. In particular, iron (Fe) was considered of great importance as early biological oceanographers hypothesized that iron may also be a limiting factor for primary production in the ocean. As a result an extended Redfield ratio was developed to include this as part of this balance. This new stoichiometric ratio states that the ratio should be 106 C:16 N:1 P:0.1-0.001 Fe. The variation in iron is the result of “…iron contamination on ships and in labs is large and difficult to control. No one has been able to beat this nearly insuperable combination of difficulties.” (Broecker and Peng (1982)). It is this contamination that resulted in early evidence suggesting that iron concentrations were high and not a limiting factor in marine primary production.
Redfield Ratio in Diatoms
Diatoms need, among other nutrients, silicic acid to create biogenic silica for their frustules (cell walls). As a result of this the Redfield-Brzezinski nutrient ratio was proposed for diatoms and stated to be C:Si:N:P = 106:15:16:1 (Brzezinski, 1985).
See also
Example
In 2014, a article was released in Nature,[3] which aggregated Redfield ratios measurements from observational cruises around the world from 1970 to 2010. This article gave a large database usable to study the evolution of particular phosphorus, carbon and nitrogen across sea stations and time.
References
- ↑ http://lgmacweb.env.uea.ac.uk/green_ocean/positions/diazotroph.shtml
- ↑ http://www.mbari.org/chemsensor/distribution.html
- ↑ Martiny, A. C., Vrugt, J. A., & Lomas, M. W. (2014). Concentrations and ratios of particulate organic carbon, nitrogen, and phosphorus in the global ocean. Scientific Data, 1., http://www.nature.com/articles/sdata201448
- Arrigo, K.R., Marine microorganisms and global nutrient cycles, Nature, Vol 437, pp. 349–355, 2005
- Brzezinski, M.A., The Si:C:N ratio of marine diatoms: interspecific variability and the effect of some environmental variables. Journal of Phycology, Vo. 21, pp. 347–357, 1985
- Johnson, Zackary. "Biogeochemistry IV." University of Hawaii School of Ocean and Earth Science and Technology. Web. <http://www.soest.hawaii.edu/oceanography/zij/ocn621/OCN621-20060215-biogeochemistry.pdf>.
- Lentz, Jennifer. "Nutrient Stoichiometry - Redfield Ratios." LSU School of the Coast and Environment, 2010. Web. <http://www.sce.lsu.edu/cego/Documents/Reviews/Oceanography/Nutrient_Stoichiometry.pdf>.
- Loladze, I., and J.J. Elser. 2011. The origins of the Redfield nitrogen-to-phosphorus ratio are in a homoeostatic protein-to-rRNA ratio. Ecology Letters, 14:244-250.
- P.G. Falkowski, and C.S. Davis. "MARINE BIOGEOCHEMISTRY: ON REDFIELD RATIOS." ScienceWeek. Nature, 2004. Web. <http://scienceweek.com/2004/sa041119-5.htm>.
- Sterner, R.W., T. Andersen, J.J. Elser, D.O. Hessen, J.M. Hood, E. McCauley, and J. Urabe. 2008. Scale-dependent carbon:nitrogen:phosphorus seston stoichiometry in marine and freshwaters. Limnology and Oceanography, 53:1169-1180.
- Redfield A.C., On the proportions of organic derivations in sea water and their relation to the composition of plankton. In James Johnstone Memorial Volume. (ed. R.J. Daniel). University Press of Liverpool, pp. 176–192, 1934.
- Redfield, A.C., The biological control of chemical factors in the environment, American Scientist, 1958