Antimicrobial resistance
Antimicrobial resistance (AMR) is the ability of a microbe to resist the effects of medication previously used to treat them.[2][3][4] This broader term also covers antibiotic resistance, which applies to bacteria and antibiotics.[3] Resistance arises through one of three ways: natural resistance in certain types of bacteria; genetic mutation; or by one species acquiring resistance from another.[5] Resistance can appear spontaneously because of random mutations; or more commonly following gradual buildup over time, and because of misuse of antibiotics or antimicrobials.[6] Resistant microbes are increasingly difficult to treat, requiring alternative medications or higher doses—which may be more costly or more toxic. Microbes resistant to multiple antimicrobials are called multidrug resistant (MDR); or sometimes superbugs.[7] Antimicrobial resistance is on the rise with millions of deaths every year.[8] A few infections are now completely untreatable because of resistance. All classes of microbes develop resistance (fungi, antifungal resistance; viruses, antiviral resistance; protozoa, antiprotozoal resistance; bacteria, antibiotic resistance).
Antibiotics should only be used when needed as prescribed by health professionals.[9] The prescriber should closely adhere to the five rights of drug administration: the right patient, the right drug, the right dose, the right route, and the right time.[10] Narrow-spectrum antibiotics are preferred over broad-spectrum antibiotics when possible, as effectively and accurately targeting specific organisms is less likely to cause resistance.[11] Cultures should be taken before treatment when indicated and treatment potentially changed based on the susceptibility report.[12][13] For people who take these medications at home, education about proper use is essential. Health care providers can minimize spread of resistant infections by use of proper sanitation: including handwashing and disinfecting between patients; and should encourage the same of the patient, visitors, and family members.[12]
Rising drug resistance can be attributed to three causes use of antibiotics: in the human population; in the animal population; and spread of resistant strains between human or non-human sources.[6] Antibiotics increase selective pressure in bacterial populations, causing vulnerable bacteria to die—this increases the percentage of resistant bacteria which continue growing. With resistance to antibiotics becoming more common there is greater need for alternative treatments. Calls for new antibiotic therapies have been issued, but new drug-development is becoming rarer.[14] There are multiple national and international monitoring programs for drug-resistant threats. Examples of drug-resistant bacteria included in this program are: methicillin-resistant Staphylococcus aureus (MRSA), vancomycin-resistant S. aureus (VRSA), extended spectrum beta-lactamase (ESBL), vancomycin-resistant Enterococcus (VRE), multidrug-resistant A. baumannii (MRAB).[15]
A World Health Organization (WHO) report released April 2014 stated, "this serious threat is no longer a prediction for the future, it is happening right now in every region of the world and has the potential to affect anyone, of any age, in any country. Antibiotic resistance—when bacteria change so antibiotics no longer work in people who need them to treat infections—is now a major threat to public health."[16] Increasing public calls for global collective action to address the threat include proposals for international treaties on antimicrobial resistance.[17] Worldwide antibiotic resistance is not fully mapped, but poorer countries with weak healthcare systems are more affected.[9] According to the Centers for Disease Control and Prevention: "Each year in the United States, at least 2 million people become infected with bacteria that are resistant to antibiotics and at least 23,000 people die each year as a direct result of these infections."[18]
Definition
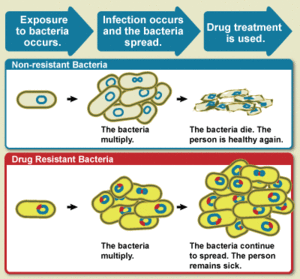
The WHO defines antimicrobial resistance as a microorganism's resistance to an antimicrobial drug that was once able to treat an infection by that microorganism.[3] A person cannot become resistant to antibiotics. Resistance is a property of the microbe, not a person or other organism infected by a microbe.[20]
Causes
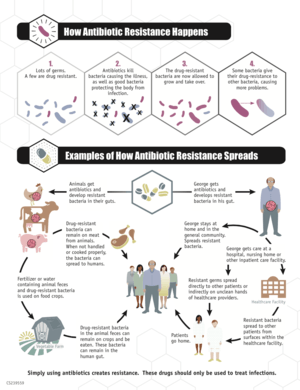
Bacteria with resistance to antibiotics predate medical use of antibiotics by humans;[21][22][23] however, widespread antibiotic use has made more bacteria resistant through the process of evolutionary pressure.[24][25]
Reasons for the widespread use of antibiotics include:
- increasing global availability over time since the 1950s
- uncontrolled sale in many low or middle income countries, where they can be obtained over the counter without a prescription, potentially resulting in antibiotics being used when not indicated.[26]:1060 This may result in emergence of resistance in any remaining bacteria.
Antibiotic use in livestock feed at low doses for growth promotion is an accepted practice in many industrialized countries and is known to lead to increased levels of resistance.[27][28] Releasing large quantities of antibiotics into the environment during pharmaceutical manufacturing through inadequate wastewater treatment increases the risk that antibiotic-resistant strains will develop and spread.[29][30] It is uncertain whether antibacterials in soaps and other products contribute to antibiotic resistance, but they are discouraged for other reasons.[31][32]
Human medicine
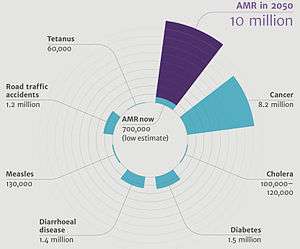
Increasing bacterial resistance is linked with the volume of antibiotic prescribed, as well as missing doses when taking antibiotics.[33] Inappropriate prescribing of antibiotics has been attributed to a number of causes, including people insisting on antibiotics, physicians prescribing them as they feel they do not have time to explain why they are not necessary, and physicians not knowing when to prescribe antibiotics or being overly cautious for medical and/or legal reasons.[34]
Up to half of antibiotics used in humans are unnecessary and inappropriate.[6] For example, a third of people believe that antibiotics are effective for the common cold,[35] and the common cold is the most common reason antibiotics are prescribed[36] even though antibiotics are useless against viruses. A single regimen of antibiotics even in compliant individuals leads to a greater risk of resistant organisms to that antibiotic in the person for a month to possibly a year.[37][38]
Antibiotic resistance increases with duration of treatment; therefore, as long as an effective minimum is kept, shorter courses of antibiotics are likely to decrease rates of resistance, reduce cost, and have better outcomes with fewer complications.[11] Short course regimens exist for community-acquired pneumonia[39] spontaneous bacterial peritonitis,[40] suspected lung infections in intense care wards,[41] so-called acute abdomen,[42] middle ear infections, sinusitis and throat infections,[43] and penetrating gut injuries.[44][45] In some situations a short course may not cure the infection as well as a long course.[46] A BMJ editorial recommended that antibiotics can often be safely stopped 72 hours after symptoms resolve.[47] Because individuals may feel better before the infection is eradicated, doctors must provide instructions to them so they know when it is safe to stop taking a prescription. Some researchers advocate doctors' using a very short course of antibiotics, reevaluating the patient after a few days, and stopping treatment if there are no clinical signs of infection.[48]
Certain antibiotic classes result in resistance more than others. Increased rates of MRSA infections are seen when using glycopeptides, cephalosporins, and quinolones.[49][50] Cephalosporins, and particularly quinolones and clindamycin, are more likely to produce colonisation with Clostridium difficile.[51][52]
Factors within the intensive care unit setting such as mechanical ventilation and multiple underlying diseases also appear to contribute to bacterial resistance.[53] Poor hand hygiene by hospital staff has been associated with the spread of resistant organisms,[54] and an increase in hand washing compliance results in decreased rates.[55]
Improper use of antibiotics can often be attributed to the presence of structural violence in particular regions. Socioeconomic factors such as race and poverty affect accessibility of and adherence to drug therapy. The efficacy of treatment programs for drug-resistant strains depends on whether or not programmatic improvements take into account the effects of structural violence.[56]
Veterinary medicine
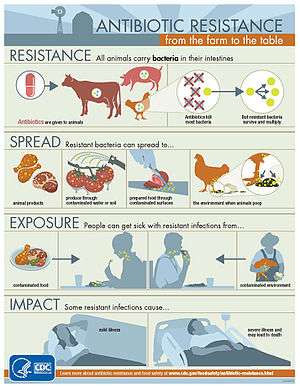
The World Health Organization concluded that inappropriate use of antibiotics in animal husbandry is an underlying contributor to the emergence and spread of antibiotic-resistant germs, and that the use of antibiotics as growth promoters in animal feeds should be restricted.[57] The World Organisation for Animal Health has added to the Terrestrial Animal Health Code a series of guidelines with recommendations to its members for the creation and harmonization of national antimicrobial resistance surveillance and monitoring programs,[58] monitoring of the quantities of antibiotics used in animal husbandry,[59] and recommendations to ensure the proper and prudent use of antibiotic substances. Another guideline is to implement methodologies that help to establish associated risk factors and assess the risk of antibiotic resistance.[60]
United States
Eighty percent of antibiotics sold in the United States are used on livestock. The majority of these antibiotics are given to animals that are otherwise healthy. Rather, it is normal practice to mix antibiotics with livestock food to promote healthier living conditions and to encourage animal growth.[61] The use of antibiotics in animals is to a large degree involved in the emergence of antibiotic-resistant microorganisms.[62] Antibiotics are used in food with the intention of not only preventing, controlling, and treating diseases, but also to promote growth.[6] Antibiotic use in animals can be classified into therapeutic, prophylactic, metaphylactic, and growth promotion uses of antibiotics.[63] All four patterns select for bacterial resistance, since antibiotic resistance is a natural evolutionary process, but the non-therapeutic uses expose larger number of animals, and therefore of bacteria, for more extended periods, and at lower doses. They therefore greatly increase the cross-section for the evolution of resistance.
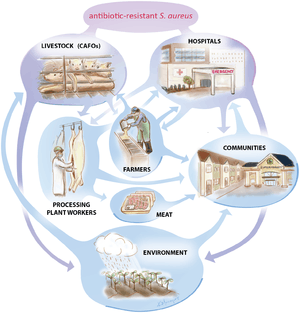
[64] Since the last third of the 20th century, antibiotics have been used extensively in animal husbandry. In 2013, 80% of antibiotics used in the US were used in animals and only 20% in humans; in 1997 half were used in humans and half in animals.[65] Some antibiotics are not used and not considered significant for use in humans, because they either lack efficacy or purpose in humans, such as ionophores in ruminants,[66] or because the drug has gone out of use in humans. Others are used in both animals and humans, including penicillin and some forms of tetracycline.[67] Historically, regulation of antibiotic use in food animals has been limited to limiting drug residues in meat, egg, and milk products, rather than by direct concern over the development of antibiotic resistance. This mirrors the primary concerns in human medicine, where, in general, researchers and doctors were more concerned about effective but non-toxic doses of drugs rather than antibiotic resistance.
In 2001, the Union of Concerned Scientists estimated that greater than 70% of the antibiotics used in the U.S. are given to food animals (for example, chickens, pigs, and cattle), in the absence of disease.[65][68] The amounts given are termed "sub-therapeutic", i.e., insufficient to combat disease. Despite no diagnosis of disease, the administration of these drugs (most of which are not significant to human medicine) results in decreased mortality and morbidity and increased growth in the animals so treated. It is theorized that sub-therapeutic dosages kills some, but not all, of the bacterial organisms in the animal — likely leaving those that are naturally antibiotic-resistant.[69] Studies have shown, however, that, in essence, the overall population levels of bacteria are unchanged; only the mix of bacteria is affected. The actual mechanism by which sub-therapeutic antibiotic feed additives serve as growth promoters is thus unclear. Some people have speculated that animals and fowl may have sub-clinical infections, which would be cured by low levels of antibiotics in feed, thereby allowing the creatures to thrive. No convincing evidence has been advanced for this theory, and the bacterial load in an animal is essentially unchanged by use of antibiotic feed additives. The mechanism of growth promotion is therefore probably something other than "killing off the bad bugs".
Antibiotics are used in U.S. animal feed to promote animal productivity.[28][70] In particular, poultry feed and water is a common route of administration of drugs, because of higher overall costs when drugs are administered by handling animals individually.
In research studies, occasional animal-to-human spread of antibiotic-resistant organisms has been demonstrated. Resistant bacteria can be transmitted from animals to humans in three ways: by consuming animal products (milk, meat, eggs, etc.), from close or direct contact with animals or other humans, or through the environment.[71] In the first pathway, food preservation methods can help eliminate, decrease, or prevent the growth of bacteria in some food classes. Evidence for the transfer of macrolide-resistant microorganisms from animals to humans has been scant, and most evidence shows that pathogens of concern in human populations originated in humans and are maintained there, with rare cases of transference to humans.[72][73]
Natural occurrence
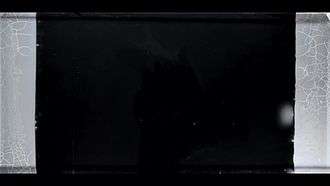
Naturally occurring antibiotic resistance is common.[75] Genes for resistance to antibiotics, like antibiotics themselves, are ancient.[76]:457–461The genes that confer resistance are known as the environmental resistome.[75] These genes may be transferred from non-disease-causing bacteria to those that do cause disease, leading to clinically significant antibiotic resistance.[75] In 1952 it was shown that penicillin-resistant bacteria existed before penicillin treatment;[77] and also preexistent bacterial resistance to streptomycin.[78] In 1962, the presence of penicillinase was detected in dormant endospores of Bacillus licheniformis, revived from dried soil on the roots of plants, preserved since 1689 in the British Museum.[79][80][81] Six strains of Clostridium, found in the bowels of William Braine and John Hartnell (members of the Franklin Expedition) showed resistance to cefoxitin and clindamycin.[82] Penicillinase may have emerged as a defense mechanism for bacteria in their habitats, such as the case of penicillinase-rich Staphylococcus aureus, living with penicillin-producing Trichophyton; however, this may be circumstantial.[81] Search for a penicillinase ancestor has focused on the class of proteins that must be a priori capable of specific combination with penicillin.[83] The resistance to cefoxitin and clindamycin in turn was attributed to Braine's and Hartnell's contact with microorganisms that naturally produce them or random mutation in the chromosomes of Clostridium strains.[82] There is evidence that heavy metals and other pollutants may select for antibiotic-resistant bacteria, generating a constant source of them in small numbers.[84]
Environmental
Antibiotic resistance is a growing problem among humans and wildlife in terrestrial or aquatic environments. In this respect, the spread and contamination of the environment, especially through "hot spots" such as hospital wastewater and untreated urban wastewater, is a growing and serious public health problem.[85] Antibiotics have been polluting the environment since their introduction through human waste (medication, farming), animals, and the pharmaceutical industry.[86] Along with antibiotic waste, resistant bacteria follow, thus introducing antibiotic-resistant bacteria into the environment. As bacteria replicate quickly, the resistant bacteria that enter the environment replicate their resistance genes as they continue to divide. In addition, bacteria carrying resistance genes have the ability to spread those genes to other species via horizontal gene transfer. Therefore, even if the specific antibiotic is no longer introduced into the environment, antibiotic-resistance genes will persist through the bacteria that have since replicated without continuous exposure.[86] Antibiotic resistance is widespread in marine vertebrates, and they may be important reservoirs of antibiotic-resistant bacteria in the marine environment.[87]
Prevention
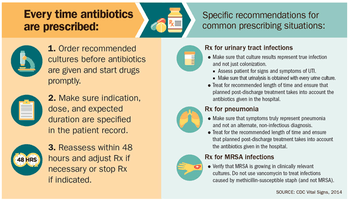
World Health Organization
- People can help tackle resistance by:
- using antibiotics only when prescribed by a doctor;
- completing the full prescription, even if they feel better;
- never sharing antibiotics with others or using leftover prescriptions.
- Health workers and pharmacists can help tackle resistance by:
- enhancing infection prevention and control;
- only prescribing and dispensing antibiotics when they are truly needed;
- prescribing and dispensing the right antibiotic(s) to treat the illness.
- Policymakers can help tackle resistance by:
- strengthening resistance tracking and laboratory capacity;
- regulating and promoting appropriate use of medicines.
- Policymakers and industry can help tackle resistance by:
- fostering innovation and research and development of new tools;
- promoting cooperation and information sharing among all stakeholders.
Duration of antibiotics
Antibiotic treatment duration should be based on the infection and other health problems a person may have. For many infections once a person has improved there is little evidence that stopping treatment causes more resistance. Some therefore feel that stopping early may be reasonable in some cases. Other infections, however, do require long courses regardless of whether a person feels better.[11]
Antibiotic usage
The Netherlands has the lowest rate of antibiotic prescribing in the OECD, at a rate of 11.4 defined daily doses (DDD) per 1,000 people per day in 2011. Germany and Sweden also have lower prescribing rates, with Sweden's rate having been declining since 2007. By contrast, Greece, France and Belgium have high prescribing rates of more than 28 DDD.[88] It is unclear if rapid viral testing affects antibiotic use in children.[89]
About a third of antibiotic prescriptions written in outpatient settings in the United States were not appropriate in 2010 and 2011. Doctors in the U.S. wrote 506 annual antibiotic scripts for every 1,000 people, with 353 being medically necessary.[90]
Monitoring
ResistanceOpen, an online global map of antimicrobial resistance developed by HealthMap, displays aggregated data on antimicrobial resistance from publicly available and user submitted data.[91][92] The website can display data for a 25-mile radius from a location. Users may submit data from antibiograms for individual hospitals or laboratories. European data is from the EARS-Net (European Antimicrobial Resistance Surveillance Network), part of the ECDC. ResistanceMap, by the Center for Disease Dynamics, Economics & Policy, also provides data on antimicrobial resistance on a global level.[93]
Strategies
Excessive antibiotic use has become one of the top contributors to the development of antibiotic resistance. Since the beginning of the antibiotic era, antibiotics have been used to treat a wide range of disease.[94] Overuse of antibiotics has become the primary cause of rising levels of antibiotic resistance. The main problem is that doctors are willing to prescribe antibiotics to ill-informed individuals who believe that antibiotics can cure nearly all illnesses, including viral infections like the common cold. In an analysis of drug prescriptions, 36% of individuals with a cold or an upper respiratory infection (both viral in origin) were given prescriptions for antibiotics.[95] These prescriptions accomplished nothing other than increasing the risk of further evolution of antibiotic resistant bacteria.
In a recent years, antimicrobial stewardship teams in hospitals have encouraged optimal use of antimicrobials.[96] The goals of antimicrobial stewardship are to help practitioners pick the right drug at the right dose and duration of therapy while preventing misuse and minimizing the development of resistance.
There have been increasing public calls for global collective action to address the threat, including a proposal for international treaty on antimicrobial resistance. Further detail and attention is still needed in order to recognize and measure trends in resistance on the international level; the idea of a global tracking system has been suggested but implementation has yet to occur. A system of this nature would provide insight to areas of high resistance as well as information necessary for evaluation of programs and other changes made to fight or reverse antibiotic resistance.
On March 27, 2015, the White House released a comprehensive plan to address the increasing need for agencies to combat the rise of antibiotic-resistant bacteria. The Task Force for Combating Antibiotic-Resistant Bacteria developed The National Action Plan for Combating Antibiotic-Resistant Bacteria with the intent of providing a roadmap to guide the US in the antibiotic resistance challenge and with hopes of saving many lives. This plan outlines steps taken by the Federal government over the next five years needed in order to prevent and contain outbreaks of antibiotic-resistant infections; maintain the efficacy of antibiotics already on the market; and to help to develop future diagnostics, antibiotics, and vaccines.[97]
The Action Plan was developed around five goals with focuses on strengthening health care, public health veterinary medicine, agriculture, food safety and research, and manufacturing. These goals, as listed by the White House, are as follows:
- Slow the Emergence of Resistant Bacteria and Prevent the Spread of Resistant Infections
- Strengthen National One-Health Surveillance Efforts to Combat Resistance
- Advance Development and use of Rapid and Innovative Diagnostic Tests for Identification and Characterization of Resistant Bacteria
- Accelerate Basic and Applied Research and Development for New Antibiotics, Other Therapeutics, and Vaccines
- Improve International Collaboration and Capacities for Antibiotic Resistance Prevention, Surveillance, Control and Antibiotic Research and Development
By following are goals set to meet by 2020:[97]
- Establishment of antimicrobial programs within acute care hospital settings
- Reduction of inappropriate antibiotic prescription and use by at least 50% in outpatient settings and 20% inpatient settings
- Establishment of State Antibiotic Resistance (AR) Prevention Programs in all 50 states
- Elimination of the use of medically important antibiotics for growth promotion in food-producing animals.
The World Health Organization has promoted the first World Antibiotic Awareness Week running from 16–22 November 2015. The aim of the week is to increase global awareness of antibiotic resistance. It also wants to promote the correct usage of antibiotics across all fields in order to prevent further instances of antibiotic resistance.[98]
Vaccines
Microorganisms do not develop resistance to vaccines because a vaccine enhances the body's immune system, whereas an antibiotic operates separately from the body's normal defenses. Furthermore, if the use of vaccines increase, there is evidence that antibiotic resistant strains of pathogens will decrease; the need for antibiotics will naturally decrease as vaccines prevent infection before it occurs.[99] However, new strains that escape immunity induced by vaccines may evolve; for example, an updated influenza vaccine is needed each year.
While theoretically promising, antistaphylococcal vaccines have shown limited efficacy, because of immunological variation between Staphylococcus species, and the limited duration of effectiveness of the antibodies produced. Development and testing of more effective vaccines is underway.[100]
Alternating therapy
Alternating therapy is a proposed method in which two or three antibiotics are taken in a rotation versus taking just one antibiotic such that bacteria resistant to one antibiotic are killed when the next antibiotic is taken. Studies have found that this method reduces the rate at which antibiotic resistant bacteria emerge in vitro relative to a single drug for the entire duration.[101]
Development of new drugs
Since the discovery of antibiotics, research and development (R&D) efforts have provided new drugs in time to treat bacteria that became resistant to older antibiotics, but in the 2000s there has been concern that development has slowed enough that seriously ill people may run out of treatment options.[102] Another concern is that doctors may become reluctant to perform routine surgeries because of the increased risk of harmful infection.[103] Backup treatments can have serious side-effects; for example, treatment of multi-drug-resistant tuberculosis can cause deafness or psychological disability.[104] The potential crisis at hand is the result of a marked decrease in industry R&D.[105] Poor financial investment in antibiotic research has exacerbated the situation.[65][105] The pharmaceutical industry has little incentive to invest in antibiotics because of the high risk and because the potential financial returns are less likely to cover the cost of development than for other pharmaceuticals.[106] In 2011, Pfizer, one of the last major pharmaceutical companies developing new antibiotics, shut down its primary research effort, citing poor shareholder returns relative to drugs for chronic illnesses.[107] However, small and medium-sized pharmaceutical companies are still active in antibiotic drug research.
In the United States, drug companies and the administration of President Barack Obama have been proposing changing the standards by which the FDA approves antibiotics targeted at resistant organisms.[103][108] On 12 December 2013, the Antibiotic Development to Advance Patient Treatment (ADAPT) Act of 2013 was introduced in the U.S. Congress. The ADAPT Act aims to fast-track the drug development in order to combat the growing public health threat of 'superbugs'. Under this Act, the FDA can approve antibiotics and antifungals needed for life-threatening infections based on data from smaller clinical trials. The Centers for Disease Control and Prevention (CDC) will reinforce the monitoring of the use of antibiotics that treat serious and life-threatening infections and the emerging resistance, and make the data publicly available. The FDA antibiotics labeling process, 'Susceptibility Test Interpretive Criteria for Microbial Organisms' or 'breakpoints' is also streamlined to allow the most up-to-date and cutting-edge data available to healthcare professionals under the new Act.[109][110]
On 18 September 2014 Obama signed an executive order[111] to implement the recommendations proposed in a report[112] by the President's Council of Advisors on Science and Technology (PCAST) which outlines strategies to stream-line clinical trials and speed up the R&D of new antibiotics. Among the proposals:
- Create a 'robust, standing national clinical trials network for antibiotic testing' which will promptly enroll patients once identified to be suffering from dangerous bacterial infections. The network will allow testing multiple new agents from different companies simultaneously for their safety and efficacy.
- Establish a 'Special Medical Use (SMU)' pathway for FDA to approve new antimicrobial agents for use in limited patient populations, shorten the approval timeline for new drug so patients with severe infections could benefit as quickly as possible.
- Provide economic incentives, especially for development of new classes of antibiotics, to offset the steep R&D costs which drive away the industry to develop antibiotics.
The executive order also included a $20 million prize to encourage the development of diagnostic tests to identify highly resistant bacterial infections.[113]
The U.S. National Institutes of Health plans to fund a new research network on the issue up to $62 million from 2013 to 2019.[114] Using authority created by the Pandemic and All Hazards Preparedness Act of 2006, the Biomedical Advanced Research and Development Authority in the U.S. Department of Health and Human Services announced that it will spend between $40 million and $200 million in funding for R&D on new antibiotic drugs under development by GlaxoSmithKline.[115]
One major cause of antibiotic resistance is the increased pumping activity of microbial ABC transporters, which diminishes the effective drug concentration inside the microbial cell. ABC transporter inhibitors that can be used in combination with current antimicrobials are being tested in clinical trials and are available for therapeutic regimens.[116]
Animal use
Europe
In 1997, European Union health ministers voted to ban avoparcin and four additional antibiotics used to promote animal growth in 1999.[117] In 2006 a ban on the use of antibiotics in European feed, with the exception of two antibiotics in poultry feeds, became effective.[118] In Scandinavia, there is evidence that the ban has led to a lower prevalence of antibiotic resistance in (nonhazardous) animal bacterial populations.[119] As of 2004, several European countries established a decline of antimicrobial resistance in humans through limiting the usage antimicrobials in agriculture and food industries without jeopardizing animal health or economic cost.[120]
United States
The United States Department of Agriculture (USDA) and the Food and Drug Administration (FDA) collect data on antibiotic use in humans and in a more limited fashion in animals.[121] The FDA first determined in 1977 that there is evidence of emergence of antibiotic-resistant bacterial strains in livestock. The long-established practice of permitting OTC sales of antibiotics (including penicillin and other drugs) to lay animal owners for administration to their own animals nonetheless continued in all states. In 2000, the FDA announced their intention to revoke approval of fluoroquinolone use in poultry production because of substantial evidence linking it to the emergence of fluoroquinolone-resistant Campylobacter infections in humans. Legal challenges from the food animal and pharmaceutical industries delayed the final decision to do so until 2006.[122] Fluroquinolones have been banned from extra-label use in food animals in the USA since 2007. However, they remain widely used in companion and exotic animals.
During 2007, two federal bills (S. 549[123] and H.R. 962[124]) aimed at phasing out "nontherapeutic" antibiotics in U.S. food animal production. The Senate bill, introduced by Sen. Edward "Ted" Kennedy, died. The House bill, introduced by Rep. Louise Slaughter, died after being referred to Committee.
In March 2012, the United States District Court for the Southern District of New York, ruling in an action brought by the Natural Resources Defense Council and others, ordered the FDA to revoke approvals for the use of antibiotics in livestock that violated FDA regulations.[125] On April 11, 2012 the FDA announced a voluntary program to phase out unsupervised use of drugs as feed additives and convert approved over-the-counter uses for antibiotics to prescription use only, requiring veterinarian supervision of their use and a prescription.[126][127] In December 2013, the FDA announced the commencement of these steps to phase out the use of antibiotics for the purposes of promoting livestock growth.[65][128]
Growing U.S. consumer concern about using antibiotics in animal feed has led to greater availability of "antibiotic-free" animal products. For example, chicken producer Perdue removed all human antibiotics from its feed and launched products labeled “antibiotic free” under the Harvestland brand in 2007. Consumer response was positive, and in 2014 Perdue also phased out ionophores from its hatchery and began using the “antibiotic free” labels on its Harvestland, Simply Smart, and Perfect Portions products.[129]
Mechanisms
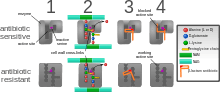
The four main mechanisms by which microorganisms exhibit resistance to antimicrobials are:
- Drug inactivation or modification: for example, enzymatic deactivation of penicillin G in some penicillin-resistant bacteria through the production of β-lactamases. Most commonly, the protective enzymes produced by the bacterial cell will add an acetyl or phosphate group to a specific site on the antibiotic, which will reduce its ability to bind to the bacterial ribosomes and disrupt protein synthesis.[130]
- Alteration of target site: for example, alteration of PBP—the binding target site of penicillins—in MRSA and other penicillin-resistant bacteria. Another protective mechanism found among bacterial species is ribosomal protection proteins. These proteins protect the bacterial cell from antibiotics that target the cell’s ribosomes to inhibit protein synthesis. The mechanism involves the binding of the ribosomal protection proteins to the ribosomes of the bacterial cell, which in turn changes its conformational shape. This allows the ribosomes to continue synthesizing proteins essential to the cell while preventing antibiotics from binding to the ribosome to inhibit protein synthesis.
- Alteration of metabolic pathway: for example, some sulfonamide-resistant bacteria do not require para-aminobenzoic acid (PABA), an important precursor for the synthesis of folic acid and nucleic acids in bacteria inhibited by sulfonamides, instead, like mammalian cells, they turn to using preformed folic acid.
- Reduced drug accumulation: by decreasing drug permeability or increasing active efflux (pumping out) of the drugs across the cell surface[131] These specialized pumps can be found within the cellular membrane of certain bacterial species and are used to pump antibiotics out of the cell before they are able to do any damage. These efflux pumps are often activated by a specific substrate associated with an antibiotic.[132]

Antibiotic resistance can be a result of horizontal gene transfer,[133] and also of unlinked point mutations in the pathogen genome at a rate of about 1 in 108 per chromosomal replication. Mutations are rare but the fact that bacteria reproduce at such a high rate allows for the effect to be significant. A mutation may produce a change in the binding site of the antibiotic, which may allow the site to continue proper functioning in the presence of the antibiotic or prevent the binding of the antibiotic to the site altogether. Research has shown the bacterial protein LexA may play a key role in the acquisition of bacterial mutations giving resistance to quinolones and rifampicin. DNA damage induces the SOS gene repressor LexA to undergo autoproteolytic activity. This includes the transcription of genes encoding Pol II, Pol IV, and Pol V, which are three nonessential DNA polymerases that are required for mutation in response to DNA damage.[134] The antibiotic action against the pathogen can be seen as an environmental pressure. Those bacteria with a mutation that allows them to survive live to reproduce. They then pass this trait to their offspring, which leads to the evolution of a fully resistant colony. Although these chromosomal mutations may seem to benefit the bacteria by providing antibiotic resistance, they also confer a cost of fitness. For example, a ribosomal mutation may protect a bacterial cell by changing the binding site of an antibiotic but it will also slow the process of protein synthesis.[130] Additionally, a particular study specifically compared the overall fitness of antibiotic resistant strains of Escherichia coli and Salmonella typhimurium to their drug-sensitive revertants. They observed a reduced overall fitness in the antibiotic resistant strains, especially in growth rate.[135]
There are three known mechanisms of fluoroquinolone resistance. Some types of efflux pumps can act to decrease intracellular quinolone concentration.[136] In Gram-negative bacteria, plasmid-mediated resistance genes produce proteins that can bind to DNA gyrase, protecting it from the action of quinolones. Finally, mutations at key sites in DNA gyrase or topoisomerase IV can decrease their binding affinity to quinolones, decreasing the drug's effectiveness.[137]
Antibiotic resistance can also be introduced artificially into a microorganism through laboratory protocols, sometimes used as a selectable marker to examine the mechanisms of gene transfer or to identify individuals that absorbed a piece of DNA that included the resistance gene and another gene of interest. A recent study demonstrated that the extent of horizontal gene transfer among Staphylococcus is much greater than previously expected—and encompasses genes with functions beyond antibiotic resistance and virulence, and beyond genes residing within the mobile genetic elements.[138]
For a long time, it has been thought that, for a microorganism to become resistant to an antibiotic, it must be in a large population. However, recent findings show that there is no necessity of large populations of bacteria for the appearance of antibiotic resistance. We know now that small populations of E.coli in an antibiotic gradient can become resistant. Any heterogeneous environment with respect to nutrient and antibiotic gradients may facilitate the development of antibiotic resistance in small bacterial populations and this is also true for the human body. Researchers hypothesize that the mechanism of resistance development is based on four SNP mutations in the genome of E.coli produced by the gradient of antibiotic. These mutations confer the bacteria emergence of antibiotic resistance.
MCR-1
In November, 2015, a Chinese team first described MCR-1 gene after finding the gene in pigs and pork. It became a matter of concern when it was discovered that it could be transferred to other organisms. MCR-1 was later discovered in Malaysia, England,[139] China,[140] Europe,[141] and the United States.[142]
NDM-1
NDM-1 is an enzyme that makes bacteria resistant to a broad range of beta-lactam antibiotics.
NDM-1 was first detected in a Klebsiella pneumoniae isolate from a Swedish patient of Indian origin in 2008. It was later detected in bacteria in India, Pakistan, the United Kingdom, the United States,[143] Canada and Japan.
According to A Lancet study, NDM-1 (New Delhi Metallo-beta-lactamase-1) originated in India, The study came to a conclusion that Indian hospitals are unsafe for treatment as Nosocomial-infections are common and with the new super-bugs on rise in India, It can be dangerous.
Organisms
Bacteria
Staphylococcus aureus
Staphylococcus aureus (colloquially known as "Staph aureus" or a "Staph infection") is one of the major resistant pathogens. Found on the mucous membranes and the human skin of around a third of the population, it is extremely adaptable to antibiotic pressure. It was one of the earlier bacteria in which penicillin resistance was found—in 1947, just four years after the drug started being mass-produced. Methicillin was then the antibiotic of choice, but has since been replaced by oxacillin because of significant kidney toxicity. Methicillin-resistant Staphylococcus aureus (MRSA) was first detected in Britain in 1961, and is now "quite common" in hospitals. MRSA was responsible for 37% of fatal cases of sepsis in the UK in 1999, up from 4% in 1991. Half of all S. aureus infections in the US are resistant to penicillin, methicillin, tetracycline and erythromycin.
This left vancomycin as the only effective agent available at the time. However, strains with intermediate (4–8 μg/ml) levels of resistance, termed glycopeptide-intermediate Staphylococcus aureus (GISA) or vancomycin-intermediate Staphylococcus aureus (VISA), began appearing in the late 1990s. The first identified case was in Japan in 1996, and strains have since been found in hospitals in England, France and the US. The first documented strain with complete (>16 μg/ml) resistance to vancomycin, termed vancomycin-resistant Staphylococcus aureus (VRSA) appeared in the United States in 2002.[144] However, in 2011, a variant of vancomycin has been tested that binds to the lactate variation and also binds well to the original target, thus reinstating potent antimicrobial activity.[145]
A new class of antibiotics, oxazolidinones, became available in the 1990s, and the first commercially available oxazolidinone, linezolid, is comparable to vancomycin in effectiveness against MRSA. Linezolid-resistance in S. aureus was reported in 2001.[146]
Community-acquired MRSA (CA-MRSA) has now emerged as an epidemic that is responsible for rapidly progressive, fatal diseases, including necrotizing pneumonia, severe sepsis, and necrotizing fasciitis.[147] MRSA is the most frequently identified antimicrobial drug-resistant pathogen in US hospitals. The epidemiology of infections caused by MRSA is rapidly changing. Since 2000, infections caused by this organism have emerged in the community. The two MRSA clones in the United States most closely associated with community outbreaks, USA400 (MW2 strain, ST1 lineage) and USA300, often contain Panton-Valentine leukocidin (PVL) genes and, more frequently, have been associated with skin and soft tissue infections. Outbreaks of CA-MRSA infections have been reported in correctional facilities, among athletic teams, among military recruits, in newborn nurseries, and among men that have sex with men. CA-MRSA infections now appear endemic in many urban regions and cause most CA-S. aureus infections.[148]
Streptococcus and Enterococcus
Streptococcus pyogenes (Group A Streptococcus: GAS) infections can usually be treated with many different antibiotics. Early treatment may reduce the risk of death from invasive group A streptococcal disease. However, even the best medical care does not prevent death in every case. For those with very severe illness, supportive care in an intensive-care unit may be needed. For persons with necrotizing fasciitis, surgery often is needed to remove damaged tissue.[149] Strains of S. pyogenes resistant to macrolide antibiotics have emerged; however, all strains remain uniformly susceptible to penicillin.[150]
Resistance of Streptococcus pneumoniae to penicillin and other beta-lactams is increasing worldwide. The major mechanism of resistance involves the introduction of mutations in genes encoding penicillin-binding proteins. Selective pressure is thought to play an important role, and use of beta-lactam antibiotics has been implicated as a risk factor for infection and colonization. S. pneumoniae is responsible for pneumonia, bacteremia, otitis media, meningitis, sinusitis, peritonitis and arthritis.[150]
Multidrug-resistant Enterococcus faecalis and Enterococcus faecium are associated with nosocomial infections.[151] These strains include: penicillin-resistant Enterococcus, vancomycin-resistant Enterococcus, and linezolid-resistant Enterococcus.[152]
Pseudomonas aeruginosa
Pseudomonas aeruginosa is a highly prevalent opportunistic pathogen. One of the most worrisome characteristics of P. aeruginosa is its low antibiotic susceptibility, which is attributable to a concerted action of multidrug efflux pumps with chromosomally encoded antibiotic resistance genes (e.g., mexAB-oprM, mexXY) and the low permeability of the bacterial cellular envelopes.[153] Pseudomonas aeruginosa has the ability to produce 4-hydroxy-2-alkylquinolines (HAQs) and it has been found that HAQs have prooxidant effects, and overexpressing modestly increased susceptibility to antibiotics. The study experimented with the Pseudomonas aeruginosa biofilms and found that a disruption of relA and spoT genes produced an inactivation of the Stringent response (SR) in cells with nutrient limitation, which provides cells be more susceptible to antibiotics.[154]
Clostridium difficile
Clostridium difficile is a nosocomial pathogen that causes diarrheal disease worldwide.[155][156] Diarrhea caused by C. difficile can be life-threatening. Infections are most frequent in people who have had recent medical and/or antibiotic treatment. C. difficile infections commonly occur during hospitalization.[15]
According to a 2015 CDC report, C. difficile caused almost 500,000 infections in the United States over a year period. Associated with these infections were an estimated 15,000 deaths. The CDC estimates that C. difficile infection costs could amount to $3.8 billion over a 5-year span.[157]
C. difficile colitis is most strongly associated with fluoroquinolones, cephalosporins, carbapenems, and clindamycin.[158][159][160]
Some research suggests the overuse of antibiotics in the raising of livestock is contributing to outbreaks of bacterial infections such as C. difficile.[16]
Antibiotics, especially those with a broad activity spectrum (such as clindamycin) disrupt normal intestinal flora. This can lead to an overgrowth of C. difficile, which flourishes under these conditions. Pseudomembranous colitis can follow, creating generalized inflammation of the colon and the development of "pseudomembrane", a viscous collection of inflammatory cells, fibrin, and necrotic cells.[4] Clindamycin-resistant C. difficile was reported as the causative agent of large outbreaks of diarrheal disease in hospitals in New York, Arizona, Florida and Massachusetts between 1989 and 1992.[161] Geographically dispersed outbreaks of C. difficile strains resistant to fluoroquinolone antibiotics, such as ciprofloxacin and levofloxacin, were also reported in North America in 2005.[162]
Carbapenem-resistant Enterobacteriaceae
As of 2013 hard-to-treat or untreatable infections of carbapenem-resistant Enterobacteriaceae (CRE) were increasing among patients in medical facilities. CRE are resistant to nearly all available antibiotics. Almost half of hospital patients who get bloodstream CRE infections die from the infection.[15]
Multidrug-resistant Acinetobacter
Acinetobacter is a gram-negative bacteria that causes pneumonia or bloodstream infections in critically ill patients. Multidrug-resistant Acinetobacter have become very resistant to antibiotics.[15]
Drug-resistant Campylobacter
Campylobacter causes diarrhea (often bloody), fever, and abdominal cramps. Serious complications such as temporary paralysis can also occur. Physicians rely on ciprofloxacin and azithromycin for treating patients with severe disease although Campylobacter is showing resistance to these antibiotics.[15]
Salmonella and E. coli
Infection with Escherichia coli and Salmonella can result from the consumption of contaminated food and water. Both of these bacteria are well known for causing nosocomial (hospital-linked) infections, and often, these strains found in hospitals are antibiotic resistant because of adaptations to wide spread antibiotic use.[163] When both bacteria are spread, serious health conditions arise. Many people are hospitalized each year after becoming infected, with some dying as a result. Since 1993, some strains of E. coli have become resistant to multiple types of fluoroquinolone antibiotics.
Although mutation alone plays a huge role in the development of antibiotic resistance, a 2008 study found that high survival rates after exposure to antibiotics could not be accounted for by mutation alone.[164] This study focused on the development of resistance in E. coli to three antibiotic drugs: ampicillin, tetracycline, and nalidixic acid. The researchers found that some antibiotic resistance in E. coli developed because of epigenetic inheritance rather than by direct inheritance of a mutated gene. This was further supported by data showing that reversion to antibiotic sensitivity was relatively common as well. This could only be explained by epigenetics.[164] Epigenetics is a type of inheritance in which gene expression is altered rather than the genetic code itself. There are many modes by which this alteration of gene expression can occur, including methylation of DNA and histone modification; however, the important point is that both inheritance of random mutations and epigenetic markers can result in the expression of antibiotic resistance genes.[164]
Resistance to polymyxins first appear in 2011.[165] An easier way for this resistance to spread, a plasmid known as MCR-1 was discovered in 2015.[165]
Acinetobacter baumannii
On November 5, 2004, the Centers for Disease Control and Prevention (CDC) reported an increasing number of Acinetobacter baumannii bloodstream infections in patients at military medical facilities in which service members injured in the Iraq/Kuwait region during Operation Iraqi Freedom and in Afghanistan during Operation Enduring Freedom were treated. Most of these showed multidrug resistance (MRAB), with a few isolates resistant to all drugs tested.[166][167]
Klebsiella pneumoniae
Klebsiella pneumoniae carbapenemase (KPC)-producing bacteria are a group of emerging highly drug-resistant Gram-negative bacilli causing infections associated with significant morbidity and mortality whose incidence is rapidly increasing in a variety of clinical settings around the world. Klebsiella pneumoniae includes numerous mechanisms for antibiotic resistance, many of which are located on highly mobile genetic elements.[168] Carbapenem antibiotics (heretofore often the treatment of last resort for resistant infections) are generally not effective against KPC-producing organisms.[169]
Mycobacterium tuberculosis
Tuberculosis is increasing across the globe, especially in developing countries, over the past few years. TB resistant to antibiotics is called MDR TB (Multidrug Resistant TB). Globally, MDR TB causes 150,000 deaths annually.[170] The rise of the HIV/AIDS epidemic has contributed to this.[171]
TB was considered one of the most prevalent diseases, and did not have a cure until the discovery of Streptomycin by Selman Waksman in 1943.[172] However, the bacteria soon developed resistance. Since then, drugs such as isoniazid and rifampin have been used. M. tuberculosis develops resistance to drugs by spontaneous mutations in its genomes. Resistance to one drug is common, and this is why treatment is usually done with more than one drug. Extensively Drug-Resistant TB (XDR TB) is TB that is also resistant to the second line of drugs.[171][173]
Resistance of Mycobacterium tuberculosis to isoniazid, rifampin, and other common treatments has become an increasingly relevant clinical challenge. (For more on Drug-Resistant TB, visit the Multi-drug-resistant tuberculosis page.) Evidence is lacking for whether these bacteria have plasmids.[174] Also M. tuberculosis lack the opportunity to interact with other bacteria in order to share plasmids.[174][175]
Neisseria gonorrhoeae
Neisseria gonorrhoeae is a sexually transmitted pathogen that causes gonorrhea, a sexually transmitted disease that can result in discharge and inflammation at the urethra, cervix, pharynx, or rectum.[15] It can cause pelvic pain, pain on urination, penile and vaginal discharge, as well as systemic symptoms. It can also cause severe reproductive complications.[15] The bacteria was first identified in 1879,[176] although some Biblical scholars believe that references to the disease can be found as early as Parshat Metzora of the Old Testament.[177]
In the 1940s effective treatment with penicillin became available, but by the 1970s resistant strains predominated. Resistance to penicillin has developed through two mechanisms: chromasomally mediated resistance (CMRNG) and penicillinase-mediated resistance (PPNG). CMRNG involves step wise mutation of penA, which codes for the penicillin-binding protein (PBP-2); mtr, which encodes an efflux pump that removes penicillin from the cell; and penB, which encodes the bacterial cell wall porins. PPNG involves the acquisition of a plasmid-borne beta-lactamase.[178] N. gonorrheoea has a high affinity for horizontal gene transfer, and as a result, the existence of any strain resistant to a given drug could spread easily across strains.
Fluoroquinolones were a useful next-line treatment until resistance was achieved through efflux pumps and mutations to the gyrA gene, which encodes DNA gyrase.[178] Third-generation cephalosporins have been used to treat gonorrhoea since 2007, but resistant strains have emerged. As of 2010, the recommended treatment is a single 250 mg intramuscular injection of ceftriaxone, sometimes in combination with azithromycin or doxycycline.[179][180] However, certain strains of N. gonorrhoeae can be resistant to antibiotics usually that are normally used to treat it. These include: cefixime (an oral cephalosporin), ceftriaxone (an injectable cephalosporin), azithromycin, aminoglycosides, and tetracycline.[15]
Viruses
Specific antiviral drugs are used to treat some viral infections. These drugs prevent viruses from reproducing by inhibiting essential stages of the virus's replication cycle in infected cells. Antivirals are used to treat HIV, hepatitis B, hepatitis C, influenza, herpes viruses including varicella zoster virus, cytomegalovirus and Epstein-Barr virus. With each virus, some strains have become resistant to the administered drugs.[181]
Resistance to HIV antivirals is problematic, and even multi-drug resistant strains have evolved.[182] Resistant strains of the HIV virus emerge rapidly if only one antiviral drug is used.[183] Using three or more drugs together has helped to control this problem, but new drugs are needed because of the continuing emergence of drug-resistant HIV strains.[184]
Fungi
Infections by fungi are a cause of high morbidity and mortality in immunocompromised persons, such as those with HIV/AIDS, tuberculosis or receiving chemotherapy.[185] The fungi candida, Cryptococcus neoformans and Aspergillus fumigatus cause most of these infections and antifungal resistance occurs in all of them.[186] Multidrug resistance in fungi is increasing because of the widespread use of antifungal drugs to treat infections in immunocompromised individuals.[187]
Of particular note, Fluconazole-resistant Candida species have been highlighted as a growing problem by the CDC.[15] More than 20 Candida species of Candida can cause Candidiasis infection, the most common of which is Candida albicans. Candida yeasts normally inhabit the skin and mucous membranes without causing infection. However, overgrowth of Candida can lead to Candidiasis. Some Candida strains are becoming resistant to first-line and second-line antifungal agents such as azoles and echinocandins.[15]
Parasites
The protozoan parasites that cause the diseases malaria, trypanosomiasis, toxoplasmosis, cryptosporidiosis and leishmaniasis are important human pathogens.[188]
Malarial parasites that are resistant to the drugs that are currently available to infections are common and this has led to increased efforts to develop new drugs.[189] Resistance to recently developed drugs such as artemisinin has also been reported. The problem of drug resistance in malaria has driven efforts to develop vaccines.[190]
Trypanosomes are parasitic protozoa that cause African trypanosomiasis and Chagas disease (American trypanosomiasis).[191][192] There are no vaccines to prevent these infections so drugs such as pentamidine and suramin, benznidazole and nifurtimox and used to treat infections. These drugs are effective but infections caused by resistant parasites have been reported.[188]
Leishmaniasis is caused by protozoa and is an important public health problem worldwide, especially in sub-tropical and tropical countries. Drug resistance has "become a major concern".[193]
Applications
Antibiotic resistance is an important tool for genetic engineering. By constructing a plasmid that contains an antibiotic-resistance gene as well as the gene being engineered or expressed, a researcher can ensure that, when bacteria replicate, only the copies that carry the plasmid survive. This ensures that the gene being manipulated passes along when the bacteria replicates.
In general, the most commonly used antibiotics in genetic engineering are "older" antibiotics. These include:
In industry, the use of antibiotic resistance is disfavored, since maintaining bacterial cultures would require feeding them large quantities of antibiotics. Instead, the use of auxotrophic bacterial strains (and function-replacement plasmids) is preferred.
Society and culture
For the fiscal year 2016 budget, President Obama has suggested to nearly double the amount of federal funding to "combat and prevent" antibiotic resistance to more than $1.2 billion.[194]
Since the mid-1980s pharmaceutical companies have invested in medications for cancer or chronic disease that have greater potential to make money and have "de-emphasized or dropped development of antibiotics".[195] On January 20, 2016 at the World Economic Forum in Davos, Switzerland, more than "80 pharmaceutical and diagnostic companies" from around the world called for 'transformational commercial models' at a global level to spur research and development on antibiotics and on the "enhanced use of diagnostic tests that can rapidly identify the infecting organism".[195]
Legal frameworks
Some global health scholars have argued that a global, legal framework is needed to prevent and control antimicrobial resistance.[196][197][17][198] For instance, binding global policies could be used to create antimicrobial use standards, regulate antibiotic marketing, and strengthen global surveillance systems.[17][196] Ensuring compliance of involved parties is a challenge.[17] Global antimicrobial resistance policies could take lessons from the environmental sector by adopting strategies that have made international environmental agreements successful in the past such as: sanctions for non-compliance, assistance for implementation, majority vote decision-making rules, an independent scientific panel, and specific commitments.[199]
See also
- Resistance-nodulation-cell division superfamily (RND)
- Alliance for the Prudent Use of Antibiotics
- Antibacterial soap
- (KPC) antibacterial resistance gene
- Broad-spectrum antibiotic
- Carbapenem-resistant enterobacteriaceae
- Colonisation resistance
- Drug of last resort
- Multidrug tolerance
- Multidrug-resistant Gram-negative bacteria
- New Delhi metallo-beta-lactamase 1
Footnotes
- ↑ Kirby-Bauer Disk Diffusion Susceptibility Test Protocol, Jan Hudzicki, ASM
- ↑ "Review on Antimicrobial Resistance". amr-review.org. Retrieved 20 May 2016.
- 1 2 3 "Antimicrobial resistance Fact sheet N°194". who.int. April 2014. Retrieved 7 March 2015.
- ↑ "About Antimicrobial Resistance, CDC
- ↑ "General Background: About Antibiotic Resistance". www.tufts.edu. Retrieved 2015-10-30.
- 1 2 3 4 "About Antimicrobial Resistance". www.cdc.gov. Retrieved 2015-10-30.
- ↑ "Antibiotic Resistance Questions & Answers". Get Smart: Know When Antibiotics Work. Centers for Disease Control and Prevention, USA. 30 June 2009. Retrieved 20 March 2013.
- ↑ WHO (April 2014). "Antimicrobial resistance: global report on surveillance 2014". WHO. WHO. Retrieved May 9, 2015.
- 1 2 Swedish work on containment of antibiotic resistance – Tools, methods and experiences (PDF). Stockholm: Public Health Agency of Sweden. 2014. pp. 16–17, 121–128. ISBN 978-91-7603-011-0.
- ↑ "The Five Rights of Medication Administration". www.ihi.org. Retrieved 2015-10-30.
- 1 2 3 "Duration of antibiotic therapy and resistance". NPS Medicinewise. National Prescribing Service Limited trading, Australia. 13 June 2013. Retrieved 22 July 2015.
- 1 2 "CDC Features – Mission Critical: Preventing Antibiotic Resistance". www.cdc.gov. Retrieved 2015-07-22.
- ↑ Leekha, Surbhi; Terrell, Christine L.; Edson, Randall S. (2011-01-01). "General Principles of Antimicrobial Therapy". Mayo Clinic Proceedings. 86 (2): 156–167. doi:10.4065/mcp.2010.0639. PMC 3031442
. PMID 21282489.
- ↑ Cassir, N; Rolain, JM; Brouqui, P (2014). "A new strategy to fight antimicrobial resistance: the revival of old antibiotics.". Frontiers in microbiology. 5: 551. doi:10.3389/fmicb.2014.00551. PMC 4202707
. PMID 25368610.
- 1 2 3 4 5 6 7 8 9 10 "Biggest Threats - Antibiotic/Antimicrobial Resistance - CDC". www.cdc.gov. Retrieved 2016-05-05.
- 1 2 "WHO's first global report on antibiotic resistance reveals serious, worldwide threat to public health" Retrieved 2014-05-02
- 1 2 3 4 Hoffman S.J.; Outterson K.; Røttingen J-A.; Cars O.; Clift C.; Rizvi Z.; Rotberg F.; Tomson G.; Zorzet A. (Feb 2015). "An International Legal Framework to Address Antimicrobial Resistance". Bulletin of the World Health Organization. 96 (2): 66. doi:10.2471/BLT.15.152710. PMID 25883395.
- ↑ https://www.cdc.gov/drugresistance/
- ↑ "What is Drug Resistance?". www.niaid.nih.gov. Retrieved 2015-07-26.
- ↑ "CDC: Get Smart: Know When Antibiotics Work". Cdc.gov. Retrieved 2013-06-12.
- ↑ D'Costa VM, King CE, Kalan L, Morar M, Sung WW, Schwarz C, Froese D, Zazula G, Calmels F, Debruyne R, Golding GB, Poinar HN, Wright GD (2011). "Antibiotic resistance is ancient". Nature. 477 (7365): 457–461. Bibcode:2011Natur.477..457D. doi:10.1038/nature10388. PMID 21881561.
- ↑ Caldwell & Lindberg 2011.
- ↑ Nelson 2009, p. 294.
- ↑ Hawkey & Jones 2009, pp. i3-i10.
- ↑ Goossens H, Ferech M, Vander Stichele R, Elseviers M (2005). "Outpatient antibiotic use in Europe and association with resistance: a cross-national database study". Lancet. 365 (9459): 579–87. doi:10.1016/S0140-6736(05)17907-0. PMID 15708101.(subscription required)
- ↑ The Lancet Infectious Diseases Commission. Antibiotic resistance—the need for global solutions. Lancet Infect Dis 2013;13: 1057–98.
- ↑ Ferber D (4 January 2002). "Livestock Feed Ban Preserves Drugs' Power". Science. 295 (5552): 27–28. doi:10.1126/science.295.5552.27a. PMID 11778017.(subscription required)
- 1 2 Mathew AG, Cissell R, Liamthong S (2007). "Antibiotic resistance in bacteria associated with food animals: a United States perspective of livestock production". Foodborne Pathog. Dis. 4 (2): 115–33. doi:10.1089/fpd.2006.0066. PMID 17600481.
- ↑ "Pharmaceuticals Sold In Sweden Cause Serious Environmental Harm In India, Research Shows". ScienceDaily. ScienceDaily, LLC. 7 February 2009. Retrieved 29 January 2015.
We estimated that the[water] treatment plant released 45 kilograms of the antibiotic ciprofloxacin in one day, which is equivalent to five times the daily consumption of Sweden,”
- ↑ Larsson DG, Fick J (Jan 2009). "Transparency throughout the production chain – a way to reduce pollution from the manufacturing of pharmaceuticals?". Regul Toxicol Pharmacol. 53 (3): 161–3. doi:10.1016/j.yrtph.2009.01.008. PMID 19545507.(subscription required)
- ↑ CDC. "Antibiotic Resistance Questions and Answers" [Are antibacterial-containing products (soaps, household cleaners, etc.) better for preventing the spread of infection? Does their use add to the problem of resistance?]. Atlanta, Georgia, USA.: Centers for Disease Control and Prevention. Retrieved February 25, 2015.
- ↑ Allison E. Aiello; Elaine L. Larson; Stuart B. Levy (2007). "Consumer Antibacterial Soaps: Effective or Just Risky?". Clinical Infectious Diseases. 45 (Supplement 2): S137–47. doi:10.1086/519255. PMID 17683018.
- ↑ Pechère JC (September 2001). "Patients' interviews and misuse of antibiotics". Clin. Infect. Dis. 33 Suppl 3: S170–3. doi:10.1086/321844. PMID 11524715.
Noncompliance may have an impact on antibiotic resistance ... Type A consists in shorter than prescribed courses. By reducing the antibiotic pressure, provided that the daily doses are otherwise respected, one may see a theoretical advantage ... Type B noncompliance reduces the number of daily doses ... indicate that such underdosing may promote the selection of resistance
- ↑ Arnold SR, Straus SE (2005). Arnold SR, ed. "Interventions to improve antibiotic prescribing practices in ambulatory care". Cochrane Database of Systematic Reviews (4): CD003539. doi:10.1002/14651858.CD003539.pub2. PMID 16235325.
- ↑ McNulty CA, Boyle P, Nichols T, Clappison P, Davey P (August 2007). "The public's attitudes to and compliance with antibiotics". J. Antimicrob. Chemother. 60 Suppl 1: i63–8. doi:10.1093/jac/dkm161. PMID 17656386.(subscription required)
- ↑ editors, Ronald Eccles, Olaf Weber, (2009). Common cold (Online-Ausg. ed.). Basel: Birkhäuser. p. 234. ISBN 978-3-7643-9894-1.
- ↑ Costelloe C, Metcalfe C, Lovering A, Mant D, Hay AD (2010). "Effect of antibiotic prescribing in primary care on antimicrobial resistance in individual patients: systematic review and meta-analysis". British Medical Journal. 340: c2096. doi:10.1136/bmj.c2096. PMID 20483949.(subscription required)
- ↑ Antimicrobial Resistance: Tackling a Crises for the Health and Welfare of Nations: 2014. Jim O'Neill Gibbs Building, 215 Euston Road, London, NW1 2BE. 11 December 2014.(subscription required)
- ↑ Li JZ, Winston LG, Moore DH, Bent S (September 2007). "Efficacy of short-course antibiotic regimens for community-acquired pneumonia: a meta-analysis". Am. J. Med. 120 (9): 783–90. doi:10.1016/j.amjmed.2007.04.023. PMID 17765048.(subscription required)
- ↑ Runyon BA, McHutchison JG, Antillon MR, Akriviadis EA, Montano AA (June 1991). "Short-course versus long-course antibiotic treatment of spontaneous bacterial peritonitis. A randomized controlled study of 100 patients". Gastroenterology. 100 (6): 1737–42. PMID 2019378.(subscription required)
- ↑ Singh N, Rogers P, Atwood CW, Wagener MM, Yu VL (1 August 2000). "Short-course Empiric Antibiotic Therapy for Patients with Pulmonary Infiltrates in the Intensive Care Unit A Proposed Solution for Indiscriminate Antibiotic Prescription". Am. J. Respir. Crit. Care Med. 162 (2): 505–511. doi:10.1164/ajrccm.162.2.9909095. PMID 10934078.(subscription required)
- ↑ Gleisner AL, Argenta R, Pimentel M, Simon TK, Jungblut CF, Petteffi L, de Souza RM, Sauerssig M, Kruel CD, Machado AR (30 April 2004). "Infective complications according to duration of antibiotic treatment in acute abdomen". International Journal of Infectious Diseases. 8 (3): 155–162. doi:10.1016/j.ijid.2003.06.003. PMID 15109590.(subscription required)
- ↑ Pichichero ME, Cohen R (1997). "Shortened course of antibiotic therapy for acute otitis media, sinusitis and tonsillopharyngitis". The Pediatric Infectious Disease Journal. 16 (7): 680–95. doi:10.1097/00006454-199707000-00011. PMID 9239773.(subscription required)
- ↑ Dellinger EP, Wertz MJ, Lennard ES, Oreskovich MR (1986). "Efficacy of Short-Course Antibiotic Prophylaxis After Penetrating Intestinal Injury". Archives of Surgery. 121 (1): 23–30. doi:10.1001/archsurg.1986.01400010029002. PMID 3942496.(subscription required)
- ↑ Perez-Gorricho B, Ripoll M (2003). "Does short-course antibiotic therapy better meet patient expectations?". International Journal of Antimicrobial Agents. 21 (3): 222–8. doi:10.1016/S0924-8579(02)00360-6. PMID 12636982.(subscription required)
- ↑ Keren R, Chan E (2002). "A Meta-analysis of Randomized, Controlled Trials Comparing Short- and Long-Course Antibiotic Therapy for Urinary Tract Infections in Children". Pediatrics. 109 (5): E70–0. doi:10.1542/peds.109.5.e70. PMID 11986476.(subscription required)
- ↑ McCormack J, Allan GM (2012). "A prescription for improving antibiotic prescribing in primary care". British Medical Journal. 344: d7955. doi:10.1136/bmj.d7955. PMID 22302779.(subscription required)
- ↑ Marc Bonten, MD; Eijkman-Winkler Institute for Medical Microbiology, Utrecht, the Netherland | Infectious Diseases, and Inflammation
- ↑ Tacconelli E, De Angelis G, Cataldo MA, Pozzi E, Cauda R (January 2008). "Does antibiotic exposure increase the risk of methicillin-resistant Staphylococcus aureus (MRSA) isolation? A systematic review and meta-analysis". J. Antimicrob. Chemother. 61 (1): 26–38. doi:10.1093/jac/dkm416. PMID 17986491.(subscription required)
- ↑ Muto CA, Jernigan JA, Ostrowsky BE, Richet HM, Jarvis WR, Boyce JM, Farr BM (May 2003). "SHEA guideline for preventing nosocomial transmission of multidrug-resistant strains of Staphylococcus aureus and enterococcus". Infect Control Hosp Epidemiol. 24 (5): 362–86. doi:10.1086/502213. PMID 12785411.
- ↑ Vonberg, Dr Ralf-Peter. "Clostridium difficile: a challenge for hospitals". European Center for Disease Prevention and Control. Institute for Medical Microbiology and Hospital Epidemiology: IHE. Archived from the original on 11 June 2009. Retrieved 27 July 2009.
- ↑ Kuijper EJ, van Dissel JT, Wilcox MH (Aug 2007). "Clostridium difficile: changing epidemiology and new treatment options". Current Opinion in Infectious Diseases. 20 (4): 376–83. doi:10.1097/QCO.0b013e32818be71d. PMID 17609596. (subscription required (help)).
- ↑ Thomas JK, Forrest A, Bhavnani SM, Hyatt JM, Cheng A, Ballow CH, Schentag JJ (March 1998). "Pharmacodynamic Evaluation of Factors Associated with the Development of Bacterial Resistance in Acutely Ill Patients during Therapy". Antimicrob. Agents Chemother. 42 (3): 521–7. PMC 105492
. PMID 9517926.(subscription required)
- ↑ Girou E, Legrand P, Soing-Altrach S, Lemire A, Poulain C, Allaire A, Tkoub-Scheirlinck L, Chai SH, Dupeyron C, Loche CM (October 2006). "Association between hand hygiene compliance and methicillin-resistant Staphylococcus aureus prevalence in a French rehabilitation hospital". Infect Control Hosp Epidemiol. 27 (10): 1128–30. doi:10.1086/507967. PMID 17006822.(subscription required)
- ↑ Swoboda SM, Earsing K, Strauss K, Lane S, Lipsett PA (February 2004). "Electronic monitoring and voice prompts improve hand hygiene and decrease nosocomial infections in an intermediate care unit". Crit. Care Med. 32 (2): 358–63. doi:10.1097/01.CCM.0000108866.48795.0F. PMID 14758148.(subscription required)
- ↑ Farmer, Paul E., Bruce Nizeye, Sara Stulac, and Salmaan Keshavjee. 2006. Structural Violence and Clinical Medicine. PLoS Medicine, 1686–1691. url=?
- ↑ http://www.who.int/mediacentre/factsheets/antibiotic-resistance/en/
- ↑ "1.6.7". Access online: OIE - World Organisation for Animal Health. www.oie.int. Retrieved 2015-11-14.
- ↑ "1.6.8". Access online: OIE - World Organisation for Animal Health. www.oie.int. Retrieved 2015-11-14.
- ↑ "1.6.9". Access online: OIE - World Organisation for Animal Health. www.oie.int. Retrieved 2015-11-14.
- ↑ "Antibiotic Resistance | NRDC". www.nrdc.org. Retrieved 2015-10-30.
- ↑ The Resistance Phenomenon in Microbes and Infectious Disease Vectors: Implications for Human Health and Strategies for Containment: Workshop Summary. Institute of Medicine (US) Forum on Emerging Infections; Knobler SL, Lemon SM, Najafi M, et al., editors. Washington (DC): National Academies Press (US); 2003.
- ↑ "Joint FAO/OIE/WHO Expert Workshop on Non-Human Antimicrobial Usage and Antimicrobial Resistance: Scientific assessment" (PDF). Archived from the original on 2004-09-26. Retrieved 2013-11-30.
- ↑ Smith, TC (February 2015). "Livestock-Associated Staphylococcus aureus: The United States Experience.". PLOS Pathogens. 11 (2): e1004564. doi:10.1371/journal.ppat.1004564. PMID 25654425.
- 1 2 3 4 Martin Khor (2014-05-18). "Why Are Antibiotics Becoming Useless All Over the World?". The Real News. Retrieved 2014-05-18.
- ↑ Hersom, Matt. "Application of Ionophores in Cattle Diets" (PDF). AN285 Department of Animal Sciences. University of Florida IFAS Extension. Retrieved 14 March 2013.
- ↑ The Editorial Board of the New York Times, May 10, 2014, The Rise of Antibiotic Resistance
- ↑ "Executive summary from the UCS report "Hogging It: Estimates of Antimicrobial Abuse in Livestock"". Union of Concerned Scientists. January 2001.
- ↑ Antibiotic Resistance - Linking Human And Animal Health: Improving Food Safety Through a One Health Approach Workshop Summary. Wegener, Henrik C. Washington (DC): National Academies Press (US); 2012.
- ↑ Sapkota AR, Lefferts LY, McKenzie S, Walker P (May 2007). "What Do We Feed to Food-Production Animals? A Review of Animal Feed Ingredients and Their Potential Impacts on Human Health". Environ. Health Perspect. 115 (5): 663–70. doi:10.1289/ehp.9760. PMC 1867957
. PMID 17520050.
- ↑ Schneider, K; Garrett, L (June 19, 2009). "Non-therapeutic Use of Antibiotics in Animal Agriculture, Corresponding Resistance Rates, and What Can be Done About It". Center for Global Development.
- ↑ Hurd HS, Doores S, Hayes D, Mathew A, Maurer J, Silley P, Singer RS, Jones RN (2004). "Public health consequences of macrolide use in food animals: a deterministic risk assessment". J. Food Prot. 67 (5): 980–92. PMID 15151237.(subscription required)
- ↑ Hurd HS, Malladi S (2008). "A stochastic assessment of the public health risks of the use of macrolide antibiotics in food animals". Risk Anal. 28 (3): 695–710. doi:10.1111/j.1539-6924.2008.01054.x. PMID 18643826.(subscription required)
- ↑ Baym, Michael; Lieberman, Tami D.; Kelsic, Eric D.; Chait, Remy; Gross, Rotem; Yelin, Idan; Kishony, Roy (2016-09-09). "Spatiotemporal microbial evolution on antibiotic landscapes". Science. 353 (6304): 1147–1151. doi:10.1126/science.aag0822. ISSN 0036-8075. PMID 27609891.
- 1 2 3 Wright GD (October 2010). "Antibiotic resistance in the environment: a link to the clinic?". Current Opinion in Microbiology. 13 (5): 589–94. doi:10.1016/j.mib.2010.08.005. PMID 20850375.(subscription required)
- ↑ D'Costa, Vanessa; King, Christine; Kalan, Lindsay; Morar, Mariya; Sung, Wilson; Schwarz, Carsten; Froese, Duane; Zazula, Grant; Calmels, Fabrice; Debruyne, Regis; Golding, G. Brian; Poinar, Hendrik N.; Wright, Gerard D. (September 2011). "Antibiotic resistance is ancient". Nature. 477 (7365): 457–461. Bibcode:2011Natur.477..457D. doi:10.1038/nature10388. PMID 21881561.
- ↑ "Mutations are random". University of California. Retrieved Aug 14, 2011.
- ↑ Richard William Nelson. Darwin, Then and Now: The Most Amazing Story in the History of Science, iUniverse, 2009, p. 294
- ↑ Wayne W. Umbreit, Advances in Applied Microbiology, vol. 11, Academic Press, 1970, p. 80
- ↑ Pollock MR (1967). "Origin and function of penicillinase: a problem in biochemical evolution". British Medical Journal. 4 (5571): 71–7. doi:10.1136/bmj.4.5571.71. PMC 1748446
. PMID 4963324.(subscription required)
- 1 2 New Scientist, Jun 8, 1972, p. 546
- 1 2 New Scientist, Feb 11, 1989, p. 34
- ↑ Pollock, p. 77
- ↑ Sieler, Claudia; Berendonk, Thomas U (December 14, 2012). "Heavy metal driven co-selection of antibiotic resistance in soil and water bodies impacted by agriculture and aquaculture". Frontiers in Microbiology. 3: 399. doi:10.3389/fmicb.2012.00399. PMC 3522115
. PMID 23248620.
- ↑ Levy, Stuart B. (2002-01-01). "Factors impacting on the problem of antibiotic resistance". Journal of Antimicrobial Chemotherapy. 49 (1): 25–30. doi:10.1093/jac/49.1.25. ISSN 0305-7453. PMID 11751763.
- 1 2 Martinez, J. L., & Olivares, J. (2012). Envrironmental Pollution By Antibiotic Resistance Genes. In P. L. Keen, & M. H. Montforts, Antimicrobial Resistance in the Environment (pp. 151- 171). Hoboken, N.J.: John Wiley & Sons.
- ↑ Moore M., Rose J.M. (2009). "Occurrence and patterns of antibiotic resistance invertebrates off the Northeastern United States coast". FEMS Microbiology Ecology. 67: 421–431. doi:10.1111/j.1574-6941.2009.00648.x.
- ↑ "Indicator: Antibiotic prescribing". QualityWatch. Nuffield Trust & Health Foundation. Retrieved 16 July 2015.
- ↑ Doan, Q; Enarson, P; Kissoon, N; Klassen, TP; Johnson, DW (Sep 15, 2014). "Rapid viral diagnosis for acute febrile respiratory illness in children in the Emergency Department.". The Cochrane database of systematic reviews. 9: CD006452. doi:10.1002/14651858.CD006452.pub4. PMID 25222468.
- ↑ Fleming-Dutra, Katherine E.; Hersh, Adam L.; Shapiro, Daniel J. (2016-05-03). "Prevalence of Inappropriate Antibiotic Prescriptions Among US Ambulatory Care Visits, 2010-2011". JAMA (journal).
- ↑ "HealthMap ResistanceOpen". HealthMap.org Boston Children’s Hospital. Retrieved 8 December 2015.
- ↑ Scales, David. "Mapping Antibiotic Resistance: Know The Germs In Your Neighborhood". WBUR. National Public Radio. Retrieved 8 December 2015.
- ↑ "ResistanceMap". Center for Disease Dynamics, Economics & Policy. Retrieved 8 December 2015.
- ↑ Andersson D, Hughes D (2011). "Persistence of antibiotic resistance in bacterial populations". FEMS Microbiol Rev. 35: 901–911. doi:10.1111/j.1574-6976.2011.00289.x.
- ↑ Gilberg K, Laouri M, Wade S, Isonaka S (2003). "Analysis of medication use patterns: apparent overuse of antibiotics and underuse of prescription drugs for asthma, depression, and CHF". J Managed Care Pharm. 9: 232–237.
- ↑ Doron, S; Davidson, LE (November 2011). "Antimicrobial stewardship.". Mayo Clinic Proceedings. 86 (11): 1113–23. doi:10.4065/mcp.2011.0358. PMC 3203003
. PMID 22033257.
- 1 2 "FACT SHEET: Obama Administration Releases National Action Plan to Combat Antibiotic-Resistant Bacteria". whitehouse.gov. Retrieved 2015-10-30.
- ↑ "World Antibiotic Awareness Week". World Health Organization.
- ↑ Mishra, Ravi P. N.; Oviedo-Orta, Ernesto; Prachi, Prachi; Rappuoli, Rino; Bagnoli, Fabio (2012-10-01). "Vaccines and antibiotic resistance". Current Opinion in Microbiology. 15 (5): 596–602. doi:10.1016/j.mib.2012.08.002. ISSN 1879-0364. PMID 22981392.
- ↑ "Immunity, Infectious Diseases, and Pandemics—What You Can Do". HomesteadSchools.com. Retrieved 2013-06-12.
- ↑ Kim S, Lieberman TD, Kishony R (2014). "Alternating antibiotic treatments constrain evolutionary paths to multidrug resistance". PNAS. 111 (40): 14494–14499. Bibcode:2014PNAS..11114494K. doi:10.1073/pnas.1409800111.
- ↑ "Annual Report of the Chief Medical Officer - Infections and the rise of antimicrobial resistance" (PDF). UK NHS. 2011. Archived from the original (PDF) on 22 June 2013.
- 1 2 "Obama Administration Seeks To Ease Approvals For Antibiotics". NPR. 4 June 2013. Retrieved 7 August 2016.
- ↑ "Moldova Grapples With Whether To Isolate TB Patients". NPR. 4 June 2013. Retrieved 7 August 2016.
- 1 2 Walsh, Fergus. "BBC News — Antibiotics resistance 'as big a risk as terrorism' – medical chief". Bbc.co.uk. Retrieved 2013-03-12.
- ↑ Nordrum, Amy (2015). "Antibiotic Resistance: Why Aren't Drug Companies Developing New Medicines to Stop Superbugs?". International Business Times.
- ↑ Gever, John (2011-02-04). "Pfizer Moves May Dim Prospect for New Antibiotics". MedPage Today. Retrieved 2013-03-12.
- ↑ Ledford H (2012). "FDA under pressure to relax drug rules". Nature. 492 (7427): 19. Bibcode:2012Natur.492...19L. doi:10.1038/492019a. PMID 23222585.
- ↑ Press Release (12 December 2013). "Green, Gingrey Introduce ADAPT Act to Safeguard Public Health". U.S. Congress.
- ↑ "Antibiotic Development to Advance Patient Treatment Act of 2013" (PDF). U.S. Congress. 12 December 2013.
- ↑ Office of the Press Secretary (18 September 2014). "Executive Order – Combating Antibiotics-Resistant Bacteria". The White House.
- ↑ President's Council of Advisors on Science and Technology (September 2014). "Report to the President on Combating Antibiotic Resistance" (PDF). PCAST.
- ↑ Mullin, Emily (19 September 2014). "Antibiotics R & D to get critical lift by executive order, Obama advisory group". fiercebiotech.com. Retrieved 22 September 2014.
- ↑ NIH to fund clinical research network on antibacterial resistance
- ↑ Press Release (22 May 2013). "HHS forms strategic alliance to develop new antibiotics Approach provides a pipeline of new drugs rather than a single medical countermeasure". Public Health Emergency, U.S. Department of Health & Human Services.
- ↑ Ponte-Sucre, A, ed. (2009). ABC Transporters in Microorganisms. Caister Academic Press. ISBN 978-1-904455-49-3.
- ↑ Casewell, M. (1 July 2003). "The European ban on growth-promoting antibiotics and emerging consequences for human and animal health". Journal of Antimicrobial Chemotherapy. 52 (2): 159–161. doi:10.1093/jac/dkg313.
- ↑ Castanon JI (2007). "History of the use of antibiotic as growth promoters in European poultry feeds". Poult. Sci. 86 (11): 2466–71. doi:10.3382/ps.2007-00249. PMID 17954599.(subscription required)
- ↑ Bengtsson B, Wierup M (2006). "Antimicrobial resistance in Scandinavia after ban of antimicrobial growth promoters". Anim. Biotechnol. 17 (2): 147–56. doi:10.1080/10495390600956920. PMID 17127526.(subscription required)
- ↑ Angulo, Frederick J; Baker, Nicole L; Olsen, Sonja J; Anderson, Alicia; Barrett, Timothy J (2004-04-01). "Antimicrobial use in agriculture: controlling the transfer of antimicrobial resistance to humans1". Seminars in Pediatric Infectious Diseases. Problems and Solutions for Antimicrobial Resistance among Pediatric Respiratory Tract and Nosocomiall Pathogens. 15 (2): 78–85. doi:10.1053/j.spid.2004.01.010.
- ↑ "GAO-11-801, Antibiotic Resistance: Agencies Have Made Limited Progress Addressing Antibiotic Use in Animals". gao.gov. Retrieved 2014-01-25.
- ↑ Nelson JM, Chiller TM, Powers JH, Angulo FJ (Apr 2007). "Fluoroquinolone-resistant Campylobacter species and the withdrawal of fluoroquinolones from use in poultry: a public health success story". Clin Infect Dis. 44 (7): 977–80. doi:10.1086/512369. PMID 17342653.
- ↑ "US Senate Bill S. 549: Preservation of Antibiotics for Medical Treatment Act of 2007".
- ↑ "Preservation of Antibiotics for Medical Treatment Act of 2007".
- ↑ John Gever (March 23, 2012). "FDA Told to Move on Antibiotic Use in Livestock". MedPage Today. Retrieved March 24, 2012.
- ↑ Gardiner Harris (April 11, 2012). "U.S. Tightens Rules on Antibiotics Use for Livestock". The New York Times. Retrieved April 12, 2012.
- ↑ "FDA's Strategy on Antimicrobial Resistance — Questions and Answers". U.S. Food and Drug Administration. April 11, 2012. Retrieved April 12, 2012.
"Judicious use" is using an antimicrobial drug appropriately and only when necessary; Based on a thorough review of the available scientific information, FDA recommends that use of medically important antimicrobial drugs in food-producing animals be limited to situations where the use of these drugs is necessary for ensuring animal health, and their use includes veterinary oversight or consultation. FDA believes that using medically important antimicrobial drugs to increase production in food-producing animals is not a judicious use.
- ↑ Tavernise, Sabrina. "F.D.A. to Phase Out Use of Some Antibiotics in Animals Raised for Meat". The New York Times. Retrieved 11 December 2013.
- ↑ Stephanie Strom (July 31, 2015). "Perdue Sharply Cuts Antibiotic Use in Chickens and Jabs at Its Rivals". The New York Times. Retrieved August 12, 2015.
- 1 2 [Criswell, Daniel. "The "Evolution" of Antibiotic Resistance." Institute for Creation Research. N.p., 2004. Web. 28 Oct. 2014.]
- ↑ Li XZ, Nikaido H (2009). "Efflux-Mediated Drug Resistance in Bacteria: an Update". Drugs. 69 (12): 1555–623. doi:10.2165/11317030-000000000-00000. PMC 2847397
. PMID 19678712.
- ↑ [RI Aminov, RI Mackie. Evolution and ecology of antibiotic resistance genes. Microbiology Letters. 8 May 2007. doi:10.1111/j.1574-6968.2007.00757.x]
- ↑ Ochiai, K.; Yamanaka, T; Kimura, K; Sawada, O (1959). "Inheritance of drug resistance (and its transfer) between Shigella strains and Between Shigella and E.coli strains". Hihon Iji Shimpor (in Japanese). 34: 1861.
- ↑ Cirz RT, Chin JK, Andes DR, de Crécy-Lagard V, Craig WA, Romesberg FE (2005). "Inhibition of Mutation and Combating the Evolution of Antibiotic Resistance". PLoS Biol. 3 (6): e176. doi:10.1371/journal.pbio.0030176. PMC 1088971
. PMID 15869329.
- ↑ BR Levin, V Perrot, Nina Walker. Compensatory Mutations, Antibiotic Resistance and the Population Genetics of Adaptive Evolution in Bacteria. Genetics March 1, 2000 vol. 154 no. 3 985–997.
- ↑ Morita Y, Kodama K, Shiota S, Mine T, Kataoka A, Mizushima T, Tsuchiya T (July 1998). "NorM, a Putative Multidrug Efflux Protein, of Vibrio parahaemolyticus and Its Homolog in Escherichia coli". Antimicrob. Agents Chemother. 42 (7): 1778–82. PMC 105682
. PMID 9661020.
- ↑ Robicsek A, Jacoby GA, Hooper DC (October 2006). "The worldwide emergence of plasmid-mediated quinolone resistance". Lancet Infect Dis. 6 (10): 629–40. doi:10.1016/S1473-3099(06)70599-0. PMID 17008172.
- ↑ Chan CX, Beiko RG, Ragan MA (August 2011). "Lateral Transfer of Genes and Gene Fragments in Staphylococcus Extends beyond Mobile Elements". J Bacteriol. 193 (15): 3964–3977. doi:10.1128/JB.01524-10. PMC 3147504
. PMID 21622749.
- ↑ Schnirring L (18 December 2015). "More MCR-1 findings lead to calls to ban ag use of colistin". University of Minnesota, Center for Infectious Disease Research and Policy. Retrieved 7 August 2016.
- ↑ Liu YY, Wang Y, Walsh TR, Yi LX, Zhang R, Spencer J, Doi Y, Tian G, Dong B, Huang X, Yu LF, Gu D, Ren H, Chen X, Lv L, He D, Zhou H, Lian Z, Liu JH, Shen J (February 2016). "Emergence of plasmid-mediated colistin resistance mechanism MCR-1 in animals and human beings in China: a microbiological and molecular biological study". Lancet Infectious Disease. 16 (2): 161–168. doi:10.1016/S1473-3099(15)00424-7.
- ↑ Maryn McKenna (3 December 2015). "Apocalypse Pig Redux: Last-Resort Resistance in Europe". Germination (Blog). Retrieved 7 August 2016.
- ↑ Parry L (27 June 2016). "Second patient in US is infected with 'superbug' resistant to ALL antibiotics". Daily Mail. Retrieved 7 August 2016.
- ↑
- ↑ Bozdogan B, Esel D, Whitener C, Browne FA, Appelbaum PC (2003). "Antibacterial susceptibility of a vancomycin-resistant Staphylococcus aureus strain isolated at the Hershey Medical Center". Journal of Antimicrobial Chemotherapy. 52 (5): 864–868. doi:10.1093/jac/dkg457. PMID 14563898.
- ↑ Xie J, Pierce JG, James RC, Okano A, Boger DL (2011). "A Redesigned Vancomycin Engineered for Dual d-Ala-d-Ala and d-Ala-d-Lac Binding Exhibits Potent Antimicrobial Activity Against Vancomycin-Resistant Bacteria". J. Am. Chem. Soc. 133 (35): 13946–9. doi:10.1021/ja207142h. PMC 3164945
. PMID 21823662.
- ↑ Tsiodras S, Gold HS, Sakoulas G, Eliopoulos GM, Wennersten C, Venkataraman L, Moellering RC, Ferraro MJ (July 2001). "Linezolid resistance in a clinical isolate of Staphylococcus aureus". The Lancet. 358 (9277): 207–208. doi:10.1016/S0140-6736(01)05410-1. PMID 11476839.
- ↑ Boyle-Vavra S, Daum RS (2007). "Community-acquired methicillin-resistant Staphylococcus aureus: the role of Panton-Valentine leukocidin". Lab. Invest. 87 (1): 3–9. doi:10.1038/labinvest.3700501. PMID 17146447.
- ↑ Maree CL, Daum RS, Boyle-Vavra S, Matayoshi K, Miller LG (2007). "Community-associated Methicillin-resistant Staphylococcus aureus Isolates and Healthcare-Associated Infections". Emerging Infect. Dis. 13 (2): 236–42. doi:10.3201/eid1302.060781. PMC 2725868
. PMID 17479885.
- ↑ CDCP (2005-10-11). "Group A Streptococcal (GAS) Disease (strep throat, necrotizing fasciitis, impetigo) – Frequently Asked Questions". Centers for Disease Control and Prevention. Archived from the original on 19 December 2007. Retrieved 2007-12-11.
- 1 2 Albrich WC, Monnet DL, Harbarth S (2004). "Antibiotic selection pressure and resistance in Streptococcus pneumoniae and Streptococcus pyogenes". Emerging Infect. Dis. 10 (3): 514–7. doi:10.3201/eid1003.030252. PMC 3322805
. PMID 15109426.
- ↑ Hidron AI, Edwards JR, Patel J, Horan TC, Sievert DM, Pollock DA, Fridkin SK (November 2008). National Healthcare Safety Network Team; Participating National Healthcare Safety Network Facilities. "NHSN annual update: antimicrobial-resistant pathogens associated with healthcare-associated infections: annual summary of data reported to the National Healthcare Safety Network at the Centers for Disease Control and Prevention, 2006–2007". Infect Control Hosp Epidemiol. 29 (11): 996–1011. doi:10.1086/591861. PMID 18947320.
- ↑ Kristich, Christopher J.; Rice, Louis B.; Arias, Cesar A. (2014-01-01). Gilmore, Michael S.; Clewell, Don B.; Ike, Yasuyoshi; Shankar, Nathan, eds. Enterococcal Infection—Treatment and Antibiotic Resistance. Boston: Massachusetts Eye and Ear Infirmary. PMID 24649502.
- ↑ Poole K (2004). "Efflux-mediated multiresistance in Gram-negative bacteria". Clinical Microbiology and Infection. 10 (1): 12–26. doi:10.1111/j.1469-0691.2004.00763.x. PMID 14706082.
- ↑ Nguyen D, Joshi-Datar A, Lepine F, Bauerle E, Olakanmi O, Beer K, McKay G, Siehnel R, Schafhauser J, Wang Y, Britigan BE, Singh PK (2011). "Active Starvation Responses Mediate Antibiotic Tolerance in Biofilms and Nutrient-Limited Bacteria". Science. 334 (6058): 982–6. Bibcode:2011Sci...334..982N. doi:10.1126/science.1211037. PMID 22096200.
- ↑ Gerding DN, Johnson S, Peterson LR, Mulligan ME, Silva J (1995). "Clostridium difficile-associated diarrhea and colitis". Infect. Control. Hosp. Epidemiol. 16 (8): 459–477. doi:10.1086/648363. PMID 7594392.
- ↑ McDonald LC (2005). "Clostridium difficile: responding to a new threat from an old enemy". Infect. Control. Hosp. Epidemiol. 26 (8): 672–5. doi:10.1086/502600. PMID 16156321.
- ↑ "CDC Press Releases". CDC. Retrieved 2016-05-05.
- ↑ Baxter R, Ray GT, Fireman BH (January 2008). "Case-control study of antibiotic use and subsequent Clostridium difficile-associated diarrhea in hospitalized patients". Infection Control and Hospital Epidemiology. 29 (1): 44–50. doi:10.1086/524320. PMID 18171186.
- ↑ Gifford AH, Kirkland KB (December 2006). "Risk factors for Clostridium difficile-associated diarrhea on an adult hematology-oncology ward". European Journal of Clinical Microbiology & Infectious Diseases. 25 (12): 751–5. doi:10.1007/s10096-006-0220-1. PMID 17072575.
- ↑ Palmore TN, Sohn S, Malak SF, Eagan J, Sepkowitz KA (August 2005). "Risk factors for acquisition of Clostridium difficile-associated diarrhea among outpatients at a cancer hospital". Infection Control and Hospital Epidemiology. 26 (8): 680–4. doi:10.1086/502602. PMID 16156323.
- ↑ Johnson S, Samore MH, Farrow KA, Killgore GE, Tenover FC, Lyras D, Rood JI, DeGirolami P, Baltch AL, Rafferty ME, Pear SM, Gerding DN (1999). "Epidemics of diarrhea caused by a clindamycin-resistant strain of Clostridium difficile in four hospitals". New England Journal of Medicine. 341 (23): 1645–1651. doi:10.1056/NEJM199911253412203. PMID 10572152.
- ↑ Loo VG, Poirier L, Miller MA, Oughton M, Libman MD, Michaud S, Bourgault AM, Nguyen T, Frenette C, Kelly M, Vibien A, Brassard P, Fenn S, Dewar K, Hudson TJ, Horn R, René P, Monczak Y, Dascal A (2005). "A predominantly clonal multi-institutional outbreak of Clostridium difficile-associated diarrhea with high morbidity and mortality". N Engl J Med. 353 (23): 2442–9. doi:10.1056/NEJMoa051639. PMID 16322602.
- ↑ Davies J, Davies D (2010). "Origins and Evolution of Antibiotic Resistance". Microbiol Mol Biol Rev. 74 (3): 417–433. doi:10.1128/MMBR.00016-10. PMC 2937522
. PMID 20805405.
- 1 2 3 Adam M, Murali B, Glenn N, Potter S (2008). "Epigenetic inheritance based evolution of antibiotic resistance in bacteria". BMC Evol. 8: 1–12. doi:10.1186/1471-2148-8-52.
- 1 2 Reardon, Sara (21 December 2015). "Spread of antibiotic-resistance gene does not spell bacterial apocalypse — yet". Nature. doi:10.1038/nature.2015.19037.
- ↑ "Acinetobacter baumannii infections among patients at military medical facilities treating injured U.S. service members, 2002–2004". MMWR Morb. Mortal. Wkly. Rep. Centers for Disease Control and Prevention (CDC). 53 (45): 1063–6. 2004. PMID 15549020.
- ↑ "Medscape abstract on Acinetobacter baumannii: Acinetobacter baumannii: An Emerging Multidrug-resistant Threat".
membership only website
- ↑ Hudson, Corey; Bent, Zachary; Meagher, Robert; Williams, Kelly (June 7, 2014). "Resistance Determinants and Mobile Genetic Elements of an NDM-1-Encoding Klebsiella pneumoniae Strain". PLoS ONE. 9: e99209. doi:10.1371/journal.pone.0099209. PMID 24905728.
- ↑ Arnold RS, Thom KA, Sharma S, Phillips M, Kristie Johnson J, Morgan DJ (2011). "Emergence of Klebsiella pneumoniae Carbapenemase-Producing Bacteria". Southern Medical Journal. 104 (1): 40–5. doi:10.1097/SMJ.0b013e3181fd7d5a. PMC 3075864
. PMID 21119555.
- ↑ "Antimicrobial Resistance Still Poses a Public Health Threat: A Conversation With Edward J. Septimus, MD, FIDSA, FACP, FSHEA, Clinical Professor of Internal Medicine at Texas A&M Health Science Center". Agency for Healthcare Research and Quality. 2013-04-17. Retrieved 2013-09-26.
- 1 2 LoBue P (2009). "Extensively drug-resistant tuberculosis". Current Opinion in Infectious Diseases. 22 (2): 167–73. doi:10.1097/QCO.0b013e3283229fab. PMID 19283912.
- ↑ Herzog H (1998). "History of Tuberculosis". Respiration. 65 (1): 5–15. doi:10.1159/000029220. PMID 9523361.
- ↑ Gao, Qian; Li, Xia (2010). "Transmission of MDR tuberculosis". Drug Discovery Today: Disease Mechanisms. 7: e61. doi:10.1016/j.ddmec.2010.09.006.
- 1 2 Zainuddin ZF, Dale JW (1990). "Does Mycobacterium tuberculosis have plasmids?". Tubercle. 71 (1): 43–9. doi:10.1016/0041-3879(90)90060-l. PMID 2115217.
- ↑ Louw GE, Warren RM, Gey van Pittius NC, McEvoy CR, Van Helden PD, Victor TC (2009). "A Balancing Act: Efflux/Influx in Mycobacterial Drug Resistance". Antimicrobial Agents and Chemotherapy. 53 (8): 3181–9. doi:10.1128/AAC.01577-08. PMC 2715638
. PMID 19451293.
- ↑ Ligon, B. Lee (October 2005). "Albert Ludwig Sigesmund Neisser: Discoverer of the Cause of Gonorrhea". Seminars in Pediatric Infectious Diseases. 16 (4): 336–341. doi:10.1053/j.spid.2005.07.001.
- ↑ Rosner, Fred (1995). Medicine in the Bible and the Talmud : selections from classical Jewish sources (Augm. ed.). Hoboken, NJ: KTAV Pub. House. ISBN 0-88125-506-8.
- 1 2 Tapsall (2001) Antimicrobial resistance in Niesseria gonorrhoeae. World Health Organization.
- ↑ Deguchi T, Nakane K, Yasuda M, Maeda S (September 2010). "Emergence and spread of drug resistant Neisseria gonorrhoeae". J. Urol. 184 (3): 851–8; quiz 1235. doi:10.1016/j.juro.2010.04.078. PMID 20643433.
- ↑ "Update to CDC's Sexually Transmitted Diseases Treatment Guidelines, 2010: Oral Cephalosporins No Longer a Recommended Treatment for Gonococcal Infections.". MMWR. Morbidity and mortality weekly report. 61 (31): 590–4. Aug 10, 2012. PMID 22874837.
- ↑ Lou, Z; Sun, Y; Rao, Z (February 2014). "Current progress in antiviral strategies.". Trends in pharmacological sciences. 35 (2): 86–102. doi:10.1016/j.tips.2013.11.006. PMID 24439476.
- ↑ Pennings, P. S. (2013). "HIV Drug Resistance: Problems and Perspectives". Infectious Disease Reports. 5 (Suppl 1): e5. doi:10.4081/idr.2013.s1.e5. PMC 3892620
. PMID 24470969.
- ↑ Ton, Q; Frenkel, L (2013). "HIV drug resistance in mothers and infants following use of antiretrovirals to prevent mother-to-child transmission". Current HIV research. 11 (2): 126–36. doi:10.2174/1570162x11311020005. PMID 23432488.
- ↑ Ebrahim, O; Mazanderani, AH (6 June 2013). "Recent developments in hiv treatment and their dissemination in poor countries.". Infectious disease reports. 5 (Suppl 1): e2. doi:10.4081/idr.2013.s1.e2. PMC 3892621
. PMID 24470966.
- ↑ Xie, J. L.; Polvi, E. J.; Shekhar-Guturja, T; Cowen, L. E. (2014). "Elucidating drug resistance in human fungal pathogens". Future Microbiology. 9 (4): 523–42. doi:10.2217/fmb.14.18. PMID 24810351.
- ↑ Srinivasan, A; Lopez-Ribot, J. L.; Ramasubramanian, A. K. (2014). "Overcoming antifungal resistance". Drug Discovery Today: Technologies. 11: 65–71. doi:10.1016/j.ddtec.2014.02.005. PMC 4031462
. PMID 24847655.
- ↑ Costa, C; Dias, PJ; Sá-Correia, I; Teixeira, MC (2014). "MFS multidrug transporters in pathogenic fungi: do they have real clinical impact?". Frontiers in physiology. 5: 197. doi:10.3389/fphys.2014.00197. PMC 4035561
. PMID 24904431.
- 1 2 Andrews, K. T.; Fisher, G; Skinner-Adams, T. S. (2014). "Drug repurposing and human parasitic protozoan diseases". International Journal for Parasitology: Drugs and Drug Resistance. 4 (2): 95–111. doi:10.1016/j.ijpddr.2014.02.002. PMC 4095053
. PMID 25057459.
- ↑ Visser, B. J.; Van Vugt, M; Grobusch, M. P. (2014). "Malaria: An update on current chemotherapy". Expert Opinion on Pharmacotherapy. 15 (15): 2219–54. doi:10.1517/14656566.2014.944499. PMID 25110058.
- ↑ Chia, W. N.; Goh, Y. S.; Rénia, L (2014). "Novel approaches to identify protective malaria vaccine candidates". Frontiers in Microbiology. 5: 586. doi:10.3389/fmicb.2014.00586. PMC 4233905
. PMID 25452745.
- ↑ Franco, J. R.; Simarro, P. P.; Diarra, A; Jannin, J. G. (2014). "Epidemiology of human African trypanosomiasis". Clinical Epidemiology. 6: 257–75. doi:10.2147/CLEP.S39728. PMC 4130665
. PMID 25125985.
- ↑ Herrera, L (2014). "Trypanosoma cruzi, the Causal Agent of Chagas Disease: Boundaries between Wild and Domestic Cycles in Venezuela.". Frontiers in public health. 2: 259. doi:10.3389/fpubh.2014.00259. PMC 4246568
. PMID 25506587.
- ↑ Mansueto, P; Seidita, A; Vitale, G; Cascio, A (2014). "Leishmaniasis in travelers: A literature review". Travel Medicine and Infectious Disease. 12 (6PA): 563–581. doi:10.1016/j.tmaid.2014.09.007. PMID 25287721.
- ↑ President’s 2016 Budget Proposes Historic Investment to Combat Antibiotic-Resistant Bacteria to Protect Public Health The White House, Office of the Press Secretary, January 27, 2015
- 1 2 Pollack, Andrew (20 January 2016). "To Fight 'Superbugs,' Drug Makers Call for Incentives to Develop Antibiotics". Davos 2016 Special Report. Davos, Switzerland: New York Times. Retrieved 24 January 2016.
- 1 2 Behdinan A.; Hoffman S.J. (2015). "Some Global Strategies for Antibiotic Resistance Require Legally Binding and Enforceable Commitments". Journal of Law, Medicine & Ethics. 43 (2).
- ↑ Hoffman S.J.; Ottersen T. (2015). "Mounting An Effective Response to Antibiotic Resistance Requires a Robust Global Accountability Framework". Journal of Law, Medicine & Ethics. 43 (2).
- ↑ Rizvi Z.; Hoffman S.J. (2015). "Effective Global Action on Antibiotic Resistance Calls for Careful Consideration of the Convening Forum". Journal of Law, Medicine & Ethics. 43 (2).
- ↑ Andresen S.; Hoffman S.J. (2015). "Much Can Be Learned about Addressing Antibiotic Resistance from Multilateral Environmental Agreements". Journal of Law, Medicine & Ethics. 43 (2).
References
- Books
- Caldwell, Roy; Lindberg, David, eds. (2011). "Understanding Evolution" [Mutations are random]. University of California Museum of Paleontology. Retrieved Aug 14, 2011.
- Nelson, Richard William (2009). Darwin, Then and Now: The Most Amazing Story in the History of Science (Self Published). iUniverse. p. 294.
- Journals
- Arias CA, Murray BE (2009). "Antibiotic-Resistant Bugs in the 21st Century — A Clinical Super-Challenge". New England Journal of Medicine. 360 (5): 439–443. doi:10.1056/NEJMp0804651. PMID 19179312.
- Goossens H, Ferech M, Vander Stichele R, Elseviers M (2005). Esac Project. "Outpatient antibiotic use in Europe and association with resistance: a cross-national database study". Lancet. Group Esac Project. 365 (9459): 579–87. doi:10.1016/S0140-6736(05)17907-0. PMID 15708101.
- Hawkey, PM; Jones, AM (September 2009). "The changing epidemiology of resistance". The Journal of antimicrobial chemotherapy. 64 Suppl 1: i3–10. doi:10.1093/jac/dkp256. PMID 19675017.
- Soulsby EJ (2005). "Resistance to antimicrobials in humans and animals: Overusing antibiotics is not the only cause and reducing use is not the only solution". British Medical Journal. 331 (7527): 1219–20. doi:10.1136/bmj.331.7527.1219. PMC 1289307
. PMID 16308360.
- "Alternatives to Antibiotics Reduce Animal Disease". Commonwealth Scientific and Industrial Research Organization. 9 Jan 2006.
External links
![]() |
Wikimedia Commons has media related to Antibiotic resistance. |
- Antimicrobial resistance at DMOZ
- Animation of Antibiotic Resistance
- CDC Guideline "Management of Multidrug-Resistant Organisms in Healthcare Settings, 2006"