Pseudomonas aeruginosa
Pseudomonas aeruginosa | |
---|---|
![]() | |
P. aeruginosa colony (right) on trypticase soy agar | |
Scientific classification | |
Kingdom: | Bacteria |
Phylum: | Proteobacteria |
Class: | Gammaproteobacteria |
Order: | Pseudomonadales |
Family: | Pseudomonadaceae |
Genus: | Pseudomonas |
Species: | P. aeruginosa |
Binomial name | |
Pseudomonas aeruginosa (Schröter 1872) Migula 1900 | |
Type strain | |
ATCC 10145 CCUG 551 | |
Synonyms | |
Bacterium aeruginosum Schroeter 1872 |
Pseudomonas aeruginosa is a common Gram-negative, rod-shaped bacterium that can cause disease in plants and animals, including humans. A species of considerable medical importance, P. aeruginosa is a prototypical "multidrug resistant (MDR) pathogen" recognised for its ubiquity, its intrinsically advanced antibiotic resistance mechanisms, and its association with serious illnesses – especially nosocomial infections such as ventilator-associated pneumonia and various sepsis syndromes.
The organism is considered opportunistic insofar as serious infection is often superimposed upon acute or chronic morbidity – most notably cystic fibrosis and traumatic burns – or found in immunocompromised individuals, but the organism does produce a range of clinically important infections in the immunocompetent and/or in situations where no pre-existing vulnerability is required e.g. hot tub folliculitis. In all infections produced by P. aeruginosa, treatment is dually complicated by the organism's resistance profile, which may lead to treatment failure and/or expose patients to untoward adverse effects from advanced antibiotic drug regimens. This dilemma is a central clinical problem in the field of antimicrobial resistance.
It is citrate, catalase, and oxidase positive. It is found in soil, water, skin flora, and most man-made environments throughout the world. It thrives not only in normal atmospheres, but also in hypoxic atmospheres, thus has colonized many natural and artificial environments. It uses a wide range of organic material for food; in animals, its versatility enables the organism to infect damaged tissues or those with reduced immunity. The symptoms of such infections are generalized inflammation and sepsis. If such colonizations occur in critical body organs, such as the lungs, the urinary tract, and kidneys, the results can be fatal.[1] Because it thrives on moist surfaces, this bacterium is also found on and in medical equipment, including catheters, causing cross-infections in hospitals and clinics. It is implicated in hot-tub rash. It is also able to decompose hydrocarbons and has been used to break down tarballs and oil from oil spills.[2] P. aeruginosa is not extremely virulent in comparison with other major pathogenic bacterial species – for example Staphylococcus aureus and Streptococcus pyogenes – though P. aeruginosa is capable of extensive colonization, and can aggregate into enduring biofilms.[3] P. aeruginosa does not fare especially well under suboptimal atmospheric conditions.
P. aeruginosa is among the Gram-negative bacilli commonly isolated from the exoskeletons and/or droppings of the cosmopolitan peridomestic cockroaches – including Periplaneta americana and Blatella germanica – which are often pervasive in households, as well as hospital settings. The importance of P. americana (and other vermin) as a potential reservoir or vector of P. aeruginosa continues to be studied.
Identification
Test | Results |
---|---|
Gram Stain | - |
Oxidase | + |
Indole Production | - |
Methyl Red | - |
Voges-Proskaeur | - |
Citrate | + |
Hydrogen Sulfide Production | - |
Urea Hydrolysis | + |
Phenylalanine Deaminase | - |
Lysine Decarboxylase | - |
Motility | + |
Gelatin Hydrolysis | + |
Acid from lactose | - |
acid from glucose | - |
acid from maltose | - |
acid from mannitol | + |
acid from sucrose | - |
nitrate reduction | + |
DNAse | - |
Lipase | + |
Pigment | + (bluish green pigmentation) |
Catalase | + |
[4]P. aeruginosa is a Gram-negative, aerobic (and at times facultatively anaerobic), bacillus with unipolar motility.[5] It has been identified as an opportunistic pathogen of both humans and plants.[6] P. aeruginosa is the type species of the genus Pseudomonas.[7]
In certain conditions, P. aeruginosa can secrete a variety of pigments, including pyocyanin (blue-green), pyoverdine (yellow-green and fluorescent), and pyorubin (red-brown). These can be used to identify the organism.[8]
P. aeruginosa is often preliminarily identified by its pearlescent appearance and grape-like or tortilla-like odor in vitro. Definitive clinical identification of P. aeruginosa often includes identifying the production of both pyocyanin and fluorescein, as well as its ability to grow at 42 °C. P. aeruginosa is capable of growth in diesel and jet fuels, where it is known as a hydrocarbon-using microorganism, causing microbial corrosion.[9] It creates dark, gellish mats sometimes improperly called "algae" because of their appearance.
Nomenclature
The word Pseudomonas means "false unit", from the Greek pseudo (Greek: ψευδο, false) and (Latin: monas, from Greek: μονος, a single unit). The stem word mon was used early in the history of microbiology to refer to germs, e.g., kingdom Monera.
The species name aeruginosa is a Latin word meaning verdigris ("copper rust"), referring to the blue-green color of laboratory cultures of the species. This blue-green pigment is a combination of two metabolites of P. aeruginosa, pyocyanin (blue) and pyoverdine (green), which impart the blue-green characteristic color of cultures. Another assertion is that the word may be derived from the Greek prefix ae- meaning "old or aged", and the suffix ruginosa means wrinkled or bumpy.[10]
The names pyocyanin and pyoverdine are from the Greek, with pyo-, meaning "pus",[11] cyanin, meaning "blue", and verdine, meaning "green". Pyoverdine in the absence of pyocyanin is a fluorescent-yellow color.

Biology
Genome
The genome of P. aeruginosa is relatively large (5.5–6.8 Mb) and encodes between 5,500 and 6,000 open reading frames, depending on the strain;[12] 5,021 genes are present across the first five genomes analyzed, with at least 70% sequence identity. This set of genes is the P. aeruginosa core genome.[13]
strain: | VRFPA04 | C3719 | PAO1 | PA14 | PACS2 |
---|---|---|---|---|---|
genome size (bp) | 6,818,030 | 6,222,097 | 6,264,404 | 6,537,648 | 6,492,423 |
ORFs | 5,939 | 5,578 | 5,571 | 5,905 | 5,676 |
Metabolism
P. aeruginosa is a facultative anaerobe, as it is well adapted to proliferate in conditions of partial or total oxygen depletion. This organism can achieve anaerobic growth with nitrate or nitrite as a terminal electron acceptor. When oxygen, nitrate, and nitrite are absent, it is able to ferment arginine and pyruvate by substrate-level phosphorylation.[14] Adaptation to microaerobic or anaerobic environments is essential for certain lifestyles of P. aeruginosa, for example, during lung infection in cystic fibrosis patients, where thick layers of lung mucus and alginate surrounding mucoid bacterial cells can limit the diffusion of oxygen. P. aeruginosa growth within the human body can be asymptomatic until the bacteria form a biofilm, which overwhelms the immune system. These biofilms are found in the lungs of cystic fibrosis patients, and a leading cause of death among them.[15][16][17][18][19]
Pathogenesis
An opportunistic, nosocomial pathogen of immunocompromised individuals, P. aeruginosa typically infects the airway, urinary tract, burns, and wounds, and also causes other blood infections.[20]
Infections | Details and common associations | High-risk groups |
---|---|---|
Pneumonia | Diffuse bronchopneumonia | Cystic fibrosis patients |
Septic shock | Associated with a purple-black skin lesion ecthyma gangrenosum | Neutropenic patients |
Urinary tract infection | Urinary tract catheterization | |
Gastrointestinal infection | Necrotising enterocolitis | Premature infants and neutropenic cancer patients |
Skin and soft tissue infections | Hemorrhage and necrosis | Burns victims and patients with wound infections |
It is the most common cause of infections of burn injuries and of the outer ear (otitis externa), and is the most frequent colonizer of medical devices (e.g., catheters). Pseudomonas can be spread by equipment that gets contaminated and is not properly cleaned or on the hands of healthcare workers.[21] Pseudomonas can, in rare circumstances, cause community-acquired pneumonias,[22] as well as ventilator-associated pneumonias, being one of the most common agents isolated in several studies.[23] Pyocyanin is a virulence factor of the bacteria and has been known to cause death in C. elegans by oxidative stress. However, salicylic acid can inhibit pyocyanin production.[24] One in ten hospital-acquired infections is from Pseudomonas. Cystic fibrosis patients are also predisposed to P. aeruginosa infection of the lungs. P. aeruginosa may also be a common cause of "hot-tub rash" (dermatitis), caused by lack of proper, periodic attention to water quality. Since these bacteria like moist environments, such as hot tubs and swimming pools, they can cause skin rash or swimmer's ear.[21] Pseudomonas is also a common cause of postoperative infection in radial keratotomy surgery patients. The organism is also associated with the skin lesion ecthyma gangrenosum. P. aeruginosa is frequently associated with osteomyelitis involving puncture wounds of the foot, believed to result from direct inoculation with P. aeruginosa via the foam padding found in tennis shoes, with diabetic patients at a higher risk.
Toxins
P. aeruginosa uses the virulence factor exotoxin A to inactivate eukaryotic elongation factor 2 via ADP-ribosylation in the host cell, much as the diphtheria toxin does. Without elongation factor 2, eukaryotic cells cannot synthesize proteins and necrotise. The release of intracellular contents induces an immunologic response in immunocompetent patients. In addition P. aeruginosa uses an exoenzyme, ExoU, which degrades the plasma membrane of eukaryotic cells, leading to lysis. Increasingly, it is becoming recognized that the iron-acquiring siderophore, pyoverdine, also functions as a toxin by removing iron from mitochondria, inflicting damage on this organelle.[25][26]
Phenazines
Phenazines are redox-active pigments produced by P. aeruginosa. These pigments are involved in quorum sensing, virulence, and iron acquisition.[27] P. aeruginosa produces several pigments all produced via a biosynthetic pathway. Pyocyanin, 1-Hydroxyphenazine, Phenazine-1-Carboxamide, 5-methylphenazine-1-carboxylic acid betaine, and Aeruginosin A. Two operons are involved in phenazine biosynthesis: phzA1B1C1D1E1F1G1 and phzA2B2C2D2E2F2G2.[28][29] These operons convert a chorismic acid to the phenazines mentioned above. Three key genes, phzH, phzM, and phzS convert phenazine-1-carboxylic acid to the phenazines mentioned above. Though phenazine biosynthesis is well studied, questions remain as to the final structure of the brown phenazine pyomelanin.
When pyocyanin biosynthesis is inhibited, a decrease in P. aeruginosa pathogenicity is observed in vitro.[29] This suggests that pyocyanin is most responsible for the initial colonization of P. aeruginosa in vivo.
Triggers
With low phosphate levels, P. aeruginosa has been found to activate from benign symbiont to express lethal toxins inside the intestinal tract and severely damage or kill the host, which can be mitigated by providing excess phosphate instead of antibiotics.[30]
Plants and invertebrates
In higher plants, P. aeruginosa induces soft rot, for example in Arabidopsis thaliana (Thale cress)[31] and Lactuca sativa (lettuce).[32][33] It is also pathogenic to invertebrate animals, including the nematode Caenorhabditis elegans,[34][35] the fruit fly Drosophila[36] and the moth Galleria mellonella.[37] The associations of virulence factors are the same for plant and animal infections.[32][38]
Quorum sensing
Regulation of gene expression can occur through cell-cell communication or quorum sensing (QS) via the production of small molecules called autoinducers. The extracellular accumulation of these molecules signals to bacteria to alter gene expression and coordinate behavior. P. aeruginosa employs three interconnected QS systems — lasRl, rhlRl, and PQS — that each produce unique signaling molecules.[39] Detection of these molecules indicates P. aeruginosa is growing as biofilm within the lungs of cystic fibrosis patients.[40] QS is known to control expression of a number of virulence factors, including the pigment pyocyanin. Another form of gene regulation that allows the bacteria to rapidly adapt to surrounding changes is through environmental signaling. Recent studies have discovered anaerobiosis can significantly impact the major regulatory circuit of QS. This important link between QS and anaerobiosis has a significant impact on production of virulence factors of this organism.[41] Garlic experimentally blocks quorum sensing in P. aeruginosa.[42]
Biofilms and treatment resistance
Biofilms of P. aeruginosa can cause chronic opportunistic infections, which are a serious problem for medical care in industrialized societies, especially for immunocompromised patients and the elderly. They often cannot be treated effectively with traditional antibiotic therapy. Biofilms seem to protect these bacteria from adverse environmental factors. P. aeruginosa can cause nosocomial infections and is considered a model organism for the study of antibiotic-resistant bacteria. Researchers consider it important to learn more about the molecular mechanisms that cause the switch from planktonic growth to a biofilm phenotype and about the role of QS in treatment-resistant bacteria such as P. aeruginosa. This should contribute to better clinical management of chronically infected patients, and should lead to the development of new drugs.[41]
Many genes and factors affect biofilm formation in P. aeruginosa. One of the main gene operons responsible for the initiation and maintaining the biofilm is the PSL operon.[43] This 15-gene operon is responsible for the cell-cell and cell-surface interactions required for cell communication. It is also responsible for the sequestering of the extracellular polymeric substance matrix. This matrix is composed of nucleic acids, amino acids, carbohydrates, and various ions. This matrix is one of the main resistance mechanisms in the biofilms of P. aeruginosa.
Cdi-GMP is a major contributor to biofilm adherent properties. This signalling molecule in high quantities makes superadherent biofilms. When suppressed, the biofilms are less adherent and easier to treat. Polysaccharide synthesis locus (PSL) and cdi-GMP form a negative feedback loop. PSL stimulates cdi-GMP production, while high cd-GMP turns on the operon and increases activity of the operon.
Recent studies have shown that the dispersed cells from P. aeruginosa biofilms have lower c-di-GMP levels and different physiologies from those of planktonic and biofilm cells.[44][45] Such dispersed cells are found to be highly virulent against macrophages and C. elegans, but highly sensitive towards iron stress, as compared with planktonic cells.[44]
Recently, scientists have been examining the possible genetic basis for P. aeruginosa resistance to antibiotics such as tobramycin. One locus identified as being an important genetic determinant of the resistance in this species is ndvB, which encodes periplasmic glucans that may interact with antibiotics and cause them to become sequestered into the periplasm. These results suggest a genetic basis exists behind bacterial antibiotic resistance, rather than the biofilm simply acting as a diffusion barrier to the antibiotic.[46]
Diagnosis
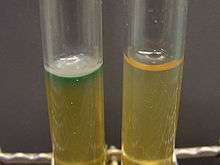
Depending on the nature of infection, an appropriate specimen is collected and sent to a bacteriology laboratory for identification. As with most bacteriological specimens, a Gram stain is performed, which may show gram-negative rods and/or white blood cells. P. aeruginosa produces colonies with a characteristic "grape-like" or "fresh-tortilla" odor on bacteriological media. In mixed cultures, it can be isolated as clear colonies on MacConkey agar (as it does not ferment lactose) which will test positive for oxidase. Confirmatory tests include production of the blue-green pigment pyocyanin on cetrimide agar and growth at 42 °C. A TSI slant is often used to distinguish nonfermenting Pseudomonas species from enteric pathogens in faecal specimens.
When P. aeruginosa is isolated from a normally sterile site (blood, bone, deep collections), it is generally considered dangerous, and almost always requires treatment. However, P. aeruginosa is frequently isolated from nonsterile sites (mouth swabs, sputum, etc.), and, under these circumstances, it may represent colonization and not infection. The isolation of P. aeruginosa from nonsterile specimens should, therefore, be interpreted cautiously, and the advice of a microbiologist or infectious diseases physician/pharmacist should be sought prior to starting treatment. Often, no treatment is needed.
Treatment
Many P. aeruginosa isolates are resistant to a large range of antibiotics and may demonstrate additional resistance after unsuccessful treatment. It should usually be possible to guide treatment according to laboratory sensitivities, rather than choosing an antibiotic empirically. If antibiotics are started empirically, then every effort should be made to obtain cultures (before administering first dose of antibiotic), and the choice of antibiotic used should be reviewed when the culture results are available.
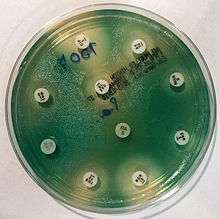
Due to widespread resistance to many common first-line antibiotics, carbapenems, polymyxins, and more recently tigecycline were considered to be the drugs of choice; however, resistance to these drugs has also been reported. Despite this, they are still being used in areas where resistance has not yet been reported. Use of β-lactamase inhibitors such as sulbactam has been advised in combination with antibiotics to enhance antimicrobial action even in the presence of a certain level of resistance. Combination therapy after rigorous antimicrobial susceptibility testing has been found to be the best course of action in the treatment of multidrug-resistant P. aeruginosa. Some next-generation antibiotics that are reported as being active against P. aeruginosa include doripenem, ceftobiprole, and ceftaroline. However, these require more clinical trials for standardization. Therefore, research for the discovery of new antibiotics and drugs against P. aeruginosa is very much needed. Antibiotics that may have activity against P. aeruginosa include:
- aminoglycosides (gentamicin, amikacin, tobramycin, but not kanamycin)
- quinolones (ciprofloxacin, levofloxacin, but not moxifloxacin)
- cephalosporins (ceftazidime, cefepime, cefoperazone, cefpirome, ceftobiprole, but not cefuroxime, cefotaxime, or ceftriaxone)
- antipseudomonal penicillins: carboxypenicillins (carbenicillin and ticarcillin), and ureidopenicillins (mezlocillin, azlocillin, and piperacillin). P. aeruginosa is intrinsically resistant to all other penicillins.
- carbapenems (meropenem, imipenem, doripenem, but not ertapenem)
- polymyxins (polymyxin B and colistin)[47]
- monobactams (aztreonam)
As fluoroquinolone is one of the few antibiotics widely effective against P. aeruginosa, in some hospitals, its use is severely restricted to avoid the development of resistant strains. On the rare occasions where infection is superficial and limited (for example, ear infections or nail infections), topical gentamicin or colistin may be used.
Antibiotic resistance
One of the most worrisome characteristics of P. aeruginosa is its low antibiotic susceptibility, which is attributable to a concerted action of multidrug efflux pumps with chromosomally encoded antibiotic resistance genes (e.g., mexAB, mexXY, etc.) and the low permeability of the bacterial cellular envelopes.[48] In addition to this intrinsic resistance, P. aeruginosa easily develops acquired resistance either by mutation in chromosomally encoded genes or by the horizontal gene transfer of antibiotic resistance determinants. Development of multidrug resistance by P. aeruginosa isolates requires several different genetic events, including acquisition of different mutations and/or horizontal transfer of antibiotic resistance genes. Hypermutation favours the selection of mutation-driven antibiotic resistance in P. aeruginosa strains producing chronic infections, whereas the clustering of several different antibiotic resistance genes in integrons favors the concerted acquisition of antibiotic resistance determinants. Some recent studies have shown phenotypic resistance associated to biofilm formation or to the emergence of small-colony variants may be important in the response of P. aeruginosa populations to antibiotics treatment.[41]
Mechanisms underlying antibiotic resistance have been found to include production of antibiotic-degrading or antibiotic-inactivating enzymes, outer membrane proteins to evict the antibiotics and mutations to change antibiotic targets. Presence of antibiotic-degrading enzymes such as extended-spectrum β-lactamases like PER-1, PER-2, VEB-1, AmpC cephalosporinases, carbapenemases like serine oxacillinases, metallo-b-lactamases, OXA-type carbapenemases, aminoglycoside-modifying enzymes, among others have been reported. P. aeruginosa can also modify the targets of antibiotic action, for example methylation of 16S rRNA to prevent aminoglycoside binding and modification of DNA, or topoisomerase to protect it from the action of quinolones. P. aeruginosa has also been reported to possess multidrug efflux pumps like AdeABC and AdeDE efflux systems that confer resistance against number of antibiotic classes. An important factor found to be associated with antibiotic resistance is the decrease in the virulence capabilities of the resistant strain. Such findings have been reported in the case of rifampicin-resistant and colistin-resistant strains, in which decrease in infective ability, quorum sensing and motility have been documented.
Mutations in DNA gyrase are commonly associated with antibiotic resistance in P. aeruginosa has. These mutations, when combined with others, confer high resistance without hindering survival. Additionally, genes involved in cyclic-di-GMP signaling may contribute to resistance. When grown in vitro conditions designed to mimic a cystic fibrosis patient's lungs, these genes mutate repeatedly.[49]
Prevention
Probiotic prophylaxis may prevent colonization and delay onset of Pseudomonas infection in an ICU setting.[50] Immunoprophylaxis against Pseudomonas is being investigated.[51] The risk of contracting 'P. aeruginosa can be reduced by avoiding pools, hot tubs, and other bodies of standing water; regularly disinfecting and/or replacing equipment that regularly encounters moisture (such as contact lens equipment and solutions); and washing one's hands often (which is protective against many other pathogens as well). However, even the best hygiene practices cannot totally protect an individual against P. aeruginosa, given how common 'P. aeruginosa is in the environment.[52]
Experimental therapies
Phage therapy against P. aeruginosa has been investigated as a possible effective treatment, which can be combined with antibiotics, has no contraindications and minimal adverse effects. Phages are produced as sterile liquid, suitable for intake, applications etc.[53] Phage therapy against ear infections caused by P. aeruginosa was reported in the journal Clinical Otolaryngology in August 2009.[54]
Research
Experimental evolution
In 2013, Joao Xavier published on an experiment in which P. aeruginosa, when subjected to repeated rounds of conditions in which it needed to swarm to acquire food, developed the ability to "hyperswarm" at speeds 25% faster than baseline organisms, by developing multiple flagella, whereas the baseline organism has a single flagellum.[55] This result was notable in the field of experimental evolution in that it was highly repeatable.[56]
See also
![]() |
Wikimedia Commons has media related to Pseudomonas aeruginosa. |
References
- ↑ Balcht, Aldona; Smith, Raymond (1994). Pseudomonas aeruginosa: Infections and Treatment. Informa Health Care. pp. 83–84. ISBN 0-8247-9210-6.
- ↑ Itah, A.Y.; Essien, J.P. (2005). "Growth Profile and Hydrocarbonoclastic Potential of Microorganisms Isolated from Tarballs in the Bight of Bonny, Nigeria". World Journal of Microbiology and Biotechnology. 21 (6–7): 1317–22. doi:10.1007/s11274-004-6694-z.
- ↑ Høiby, N; Ciofu, O,; Bjarnsholt, T. (November 2010). "Pseudomonas aeruginosa biofilms in cystic fibrosis". Future Microbiology. PubMed. 5 (11): 1663–74. doi:10.2217/fmb.10.125. PMID 21133688.
- ↑ Shovarani, Debanada (2008). "Isolation and Characterization of Pseudomonas Aeruginosa Strain DN1 Degrading p-Nitrophenol". Research Journal of Microbiology: 345–351 – via PubMed.
- ↑ Ryan, KJ; Ray, CG, eds. (2004). Sherris Medical Microbiology (4th ed.). McGraw Hill. ISBN 0-8385-8529-9.
- ↑ Iglewski BH (1996). "Pseudomonas". In Baron, S; et al. Baron's Medical Microbiology (4th ed.). University of Texas Medical Branch. ISBN 0-9631172-1-1.
- ↑ Anzai Y, Kim H, Park JY, Wakabayashi H, Oyaizu H (July 2000). "Phylogenetic affiliation of the pseudomonads based on 16S rRNA sequence". Int J Syst Evol Microbiol. 50 (4): 1563–89. doi:10.1099/00207713-50-4-1563. PMID 10939664.
- ↑ King EO, Ward MK, Raney DE (1954). "Two simple media for the demonstration of pyocyanin and fluorescein". J Lab Clin Med. 44 (2): 301–7. PMID 13184240.
- ↑ Striebich, Richard C.; Smart, Caitlin E.; Gunasekera, Thusitha S.; Mueller, Susan S.; Strobel, Ellen M.; McNichols, Brett W.; Ruiz, Oscar N. (September 2014). "Characterization of the F-76 diesel and Jet-A aviation fuel hydrocarbon degradation profiles of Pseudomonas aeruginosa and Marinobacter hydrocarbonoclasticus". International Biodeterioration & Biodegradation. 93: 33–43. doi:10.1016/j.ibiod.2014.04.024.
- ↑ Brown, RW (1956). Composition of Scientific Words. Smithsonian Institutional Press. ISBN 0-87474-286-2.
- ↑ Tzouchas, Athanase (Tom) (2014). WestBow Press. Greek Words. p. 550. ISBN 978-1490726106.
- ↑ Klockgether J, Cramer N, Wiehlmann L, Davenport CF, Tümmler B (2011). "Pseudomonas aeruginosa Genomic Structure and Diversity". Frontiers in Microbiology. 2: 150. doi:10.3389/fmicb.2011.00150. PMC 3139241
. PMID 21808635.
- ↑ Mathee K, Narasimhan G, Valdes C, Qiu X, Matewish JM, Koehrsen M, Rokas A, Yandava CN, Engels R, Zeng E, Olavarietta R, Doud M, Smith RS, Montgomery P, White JR, Godfrey PA, Kodira C, Birren B, Galagan JE, Lory S (2008). "Dynamics of Pseudomonas aeruginosa genome evolution". Proc. Natl. Acad. Sci. U.S.A. 105 (8): 3100–5. doi:10.1073/pnas.0711982105. PMC 2268591
. PMID 18287045.
- ↑ Schobert, Max; Jahn, Dieter (December 2010). "Anaerobic physiology of Pseudomonas aeruginosa in the cystic fibrosis lung". International Journal of Medical Microbiology. 300 (8): 549–556. doi:10.1016/j.ijmm.2010.08.007. PMID 20951638.
- ↑ Gerard,Funke, Case (2016). Microbiology: An Introduction (12th ed.). Pearson Education. p. 54. ISBN 978-0-321-92915-0.
- ↑ Hassett DJ (1996). "Anaerobic production of alginate by Pseudomonas aeruginosa: alginate restricts diffusion of oxygen". J. Bacteriol. 178 (24): 7322–5. PMC 178651
. PMID 8955420.
- ↑ Worlitzsch D, Tarran R, Ulrich M, Schwab U, Cekici A, Meyer KC, Birrer P, Bellon G, Berger J, Weiss T, Botzenhart K, Yankaskas JR, Randell S, Boucher RC, Döring G (2002). "Effects of reduced mucus oxygen concentration in airway Pseudomonas infections of cystic fibrosis patients". J. Clin. Invest. 109 (3): 317–325. doi:10.1172/JCI13870. PMC 150856
. PMID 11827991.
- ↑ Cooper M, Tavankar GR, Williams HD (2003). "Regulation of expression of the cyanide-insensitive terminal oxidase in Pseudomonas aeruginosa". Microbiology. 149 (Pt 5): 1275–84. doi:10.1099/mic.0.26017-0. PMID 12724389.
- ↑ Williams HD, Zlosnik JE, Ryall B (2007). "Oxygen, cyanide and energy generation in the cystic fibrosis pathogen Pseudomonas aeruginosa". Adv. Microb. Physiol. Advances in Microbial Physiology. 52: 1–71. doi:10.1016/S0065-2911(06)52001-6. ISBN 9780120277520. PMID 17027370.
- ↑ Todar's Online Textbook of Bacteriology. Textbookofbacteriology.net (2004-06-04). Retrieved on 2011-10-09.
- 1 2 "Pseudomonas aeruginosa in Healthcare Settings". Healthcare-associated Infections (HAI): Diseases and Organisms. Centers for Disease Control and Prevention. 7 May 2014.
- ↑ Fine MJ, Smith MA, Carson CA, Mutha SS, Sankey SS, Weissfeld LA, Kapoor WN (1996). "Prognosis and outcomes of patients with community-acquired pneumonia. A meta-analysis". JAMA. 275 (2): 134–141. doi:10.1001/jama.275.2.134. PMID 8531309.
- ↑ Diekema DJ, Pfaller MA, Jones RN, Doern GV, Winokur PL, Gales AC, Sader HS, Kugler K, Beach M (1999). "Survey of bloodstream infections due to gram-negative bacilli: frequency of occurrence and antimicrobial susceptibility of isolates collected in the United States, Canada, and Latin America for the SENTRY Antimicrobial Surveillance Program, 1997". Clin. Infect. Dis. 29 (3): 595–607. doi:10.1086/598640. PMID 10530454.
- ↑ Prithiviraj B, Bais HP, Weir T, Suresh B, Najarro EH, Dayakar BV, Schweizer HP, Vivanco JM (2005). "Down regulation of virulence factors of Pseudomonas aeruginosa by salicylic acid attenuates its virulence on Arabidopsis thaliana and Caenorhabditis elegans". Infect Immun. 73 (9): 5319–28. doi:10.1128/IAI.73.9.5319-5328.2005. PMC 1231131
. PMID 16113247.
- ↑ Kirienko, Natalia V.; Ausubel, Frederick M.; Ruvkun, Gary (2015-02-10). "Mitophagy confers resistance to siderophore-mediated killing by Pseudomonas aeruginosa". Proc. Natl. Acad. Sci. U.S.A. 112 (6): 1821–6. doi:10.1073/pnas.1424954112. PMID 25624506.
- ↑ Kirienko, Natalia V.; Kirienko, Daniel R.; Larkins-Ford, Jonah; Wählby, Carolina; Ruvkun, Gary; Ausubel, Frederick M. (2013-04-17). "Pseudomonas aeruginosa disrupts Caenorhabditis elegans iron homeostasis, causing a hypoxic response and death". Cell Host & Microbe. 13 (4): 406–416. doi:10.1016/j.chom.2013.03.003. ISSN 1934-6069. PMID 23601103.
- ↑ Dietrich LE, Price-Whelan A, Petersen A, Whiteley M, Newman DK (2006). "The phenazine pyocyanin is a terminal signalling factor in the quorum sensing network of Pseudomonas aeruginosa". Mol. Microbiol. 61 (5): 1308–21. doi:10.1111/j.1365-2958.2006.05306.x. PMID 16879411.
- ↑ Abu EA, Su S, Sallans L, Boissy RE, Greatens A, Heineman WR, Hassett DJ (2013). "Cyclic voltammetric, fluorescence and biological analysis of purified aeruginosin A, a secreted red pigment of Pseudomonas aeruginosa PAO1". Microbiology (Reading, Engl.). 159 (Pt 8): 1736–47. doi:10.1099/mic.0.065235-0. PMID 23782801.
Mavrodi DV, Bonsall RF, Delaney SM, Soule MJ, Phillips G, Thomashow LS (2001). "Functional analysis of genes for biosynthesis of pyocyanin and phenazine-1-carboxamide from Pseudomonas aeruginosa PAO1". J. Bacteriol. 183 (21): 6454–65. doi:10.1128/JB.183.21.6454-6465.2001. PMC 100142. PMID 11591691.
- 1 2 Ho Sui SJ, Lo R, Fernandes AR, Caulfield MD, Lerman JA, Xie L, Bourne PE, Baillie DL, Brinkman FS (2012). "Raloxifene attenuates Pseudomonas aeruginosa pyocyanin production and virulence". Int. J. Antimicrob. Agents. 40 (3): 246–51. doi:10.1016/j.ijantimicag.2012.05.009. PMID 22819149.
- ↑ "Research could lead to new non-antibiotic drugs to counter hospital infections" (Press release). University of Chicago Medical Center. 2009-04-14. Retrieved 2010-01-18.
- ↑ Walker TS, Bais HP, Déziel E, Schweizer HP, Rahme LG, Fall R, Vivanco JM (2004). "Pseudomonas aeruginosa-plant root interactions. Pathogenicity, biofilm formation, and root exudation". Plant Physiol. 134 (1): 320–331. doi:10.1104/pp.103.027888. PMC 316311
. PMID 14701912.
- 1 2 Rahme LG, Stevens EJ, Wolfort SF, Shao J, Tompkins RG, Ausubel FM (1995). "Common virulence factors for bacterial pathogenicity in plants and animals". Science. 268 (5219): 1899–1902. doi:10.1126/science.7604262. PMID 7604262.
- ↑ Rahme LG, Tan MW, Le L, Wong SM, Tompkins RG, Calderwood SB, Ausubel FM (1997). "Use of model plant hosts to identify Pseudomonas aeruginosa virulence factors". Proc. Natl. Acad. Sci. U.S.A. 94 (24): 13245–50. doi:10.1073/pnas.94.24.13245. PMC 24294
. PMID 9371831.
- ↑ Mahajan-Miklos S, Tan MW, Rahme LG, Ausubel FM (1999). "Molecular mechanisms of bacterial virulence elucidated using a Pseudomonas aeruginosa-Caenorhabditis elegans pathogenesis model". Cell. 96 (1): 47–56. doi:10.1016/S0092-8674(00)80958-7. PMID 9989496.
- ↑ Martínez C, Pons E, Prats G, León J (2004). "Salicylic acid regulates flowering time and links defence responses and reproductive development". Plant J. 37 (2): 209–17. doi:10.1046/j.1365-313X.2003.01954.x. PMID 14690505.
- ↑ D'Argenio DA, Gallagher LA, Berg CA, Manoil C (2001). "Drosophila as a model host for Pseudomonas aeruginosa infection". J. Bacteriol. 183 (4): 1466–71. doi:10.1128/JB.183.4.1466-1471.2001. PMC 95024
. PMID 11157963.
- ↑ Miyata S, Casey M, Frank DW, Ausubel FM, Drenkard E (2003). "Use of the Galleria mellonella caterpillar as a model host to study the role of the type III secretion system in Pseudomonas aeruginosa pathogenesis". Infect. Immun. 71 (5): 2404–13. doi:10.1128/IAI.71.5.2404-2413.2003. PMC 153283
. PMID 12704110.
- ↑ Rahme LG, Ausubel FM, Cao H, Drenkard E, Goumnerov BC, Lau GW, Mahajan-Miklos S, Plotnikova J, Tan MW, Tsongalis J, Walendziewicz CL, Tompkins RG (2000). "Plants and animals share functionally common bacterial virulence factors". Proc. Natl. Acad. Sci. U.S.A. 97 (16): 8815–21. doi:10.1073/pnas.97.16.8815. PMC 34017
. PMID 10922040.
- ↑ Allesen-Holm, Marie (2006). "A characterization of DNA release in Pseudomonas aeruginosa cultures and biofilms" (PDF). Molecular Microbiology.
- ↑ Winstanley C, Fothergill JL (2009). "The role of quorum sensing in chronic cystic fibrosis Pseudomonas aeruginosa infections". FEMS Microbiol. Lett. 290 (1): 1–9. doi:10.1111/j.1574-6968.2008.01394.x. PMID 19016870.
- 1 2 3 Cornelis P (editor). (2008). Pseudomonas: Genomics and Molecular Biology (1st ed.). Caister Academic Press. ISBN 1-904455-19-0.
- ↑ Bjarnsholt T, Jensen P, Rasmussen TB, Christophersen L, Calum H, Hentzer M, Hougen HP, Rygaard J, Moser C, Eberl L, Høiby N, Givskov M (2005). "Garlic blocks quorum sensing and promotes rapid clearing of pulmonary Pseudomonas aeruginosa infections". Microbiology. 151 (4): 3873–80. doi:10.1099/mic.0.27955-0. PMID 16339933.
- ↑ Colvin et al., 2013
- 1 2 Chua SL, Liu Y, Yam JK, Chen Y, Vejborg RM, Tan BG, Kjelleberg S, Tolker-Nielsen T, Givskov M, Yang L (2014). "Dispersed cells represent a distinct stage in the transition from bacterial biofilm to planktonic lifestyles". Nat Commun. 5: 4462. doi:10.1038/ncomms5462. PMID 25042103.
- ↑ Chua SL, Hultqvist LD, Yuan M, Rybtke M, Nielsen TE, Givskov M, Tolker-Nielsen T, Yang L (2015). "In vitro and in vivo generation and characterization of Pseudomonas aeruginosa biofilm-dispersed cells via c-di-GMP manipulation". Nat Protoc. 10 (8): 1165–80. doi:10.1038/nprot.2015.067. PMID 26158442.
- ↑ Mah T. F.; Pitts B.; Pellock B.; Walker G. C.; Stewart P. S.; O'Toole G. A. (2003). "A genetic basis for Pseudomonas aeruginosa biofilm antibiotic resistance". Nature. 426 (6964): 306–310. doi:10.1038/nature02122. PMID 14628055.
- ↑ Hachem RY, Chemaly RF, Ahmar CA, Jiang Y, Boktour MR, Rjaili GA, Bodey GP, Raad II (2007). "Colistin is effective in treatment of infections caused by multidrug-resistant Pseudomonas aeruginosa in cancer patients". Antimicrob. Agents Chemother. 51 (6): 1905–11. doi:10.1128/AAC.01015-06. PMC 1891378
. PMID 17387153.
- ↑ Poole K (2004). "Efflux-mediated multiresistance in gram-negative bacteria". Clinical Microbiology and Infection. 10 (1): 12–26. doi:10.1111/j.1469-0691.2004.00763.x. PMID 14706082.
- ↑ Wong, Alex; Rodrigue, Nicolas; Kassen, Rees (13 September 2012). "Genomics of Adaptation during Experimental Evolution of the Opportunistic Pathogen Pseudomonas aeruginosa". PLoS Genetics. 8 (9): e1002928. doi:10.1371/journal.pgen.1002928. PMC 3441735
. PMID 23028345.
- ↑ Forestier C, Guelon D, Cluytens V, Gillart T, Sirot J, De Champs C (2008). "Oral probiotic and prevention of Pseudomonas aeruginosa infections: a randomized, double-blind, placebo-controlled pilot study in ICU-patients". Crit Care. 12 (3): R69. doi:10.1186/cc6907. PMC 2481460
. PMID 18489775.
- ↑ Döring G, Pier GB (2008). "Vaccines and immunotherapy against Pseudomonas aeruginosa". Vaccine. 26 (8): 1011–24. doi:10.1016/j.vaccine.2007.12.007. PMID 18242792.
- ↑ http://www.childrenshospitalofillinois.org/pdfs/specialty-services/cf/germs-infection-control/Pseudomonas-Aeurigonsa-Information-Sheet.pdf
- ↑ Sulakvelidze A, Alavidze Z, Morris JG (2001). "Bacteriophage therapy". Antimicrobial Agents and Chemotherapy. 45 (3): 649–659. doi:10.1128/AAC.45.3.649-659.2001. PMC 90351
. PMID 11181338.
- ↑ Wright A, Hawkins CH, Anggård EE, Harper DR (2009). "A controlled clinical trial of a therapeutic bacteriophage preparation in chronic otitis due to antibiotic-resistant Pseudomonas aeruginosa; a preliminary report of efficacy". Clinical Otolaryngology. 34 (4): 349–357. doi:10.1111/j.1749-4486.2009.01973.x. PMID 19673983.
- ↑ van Ditmarsch D, Boyle KE, Sakhtah H, Oyler JE, Nadell CD, Déziel É, Dietrich LE, Xavier JB (2013). "Convergent evolution of hyperswarming leads to impaired biofilm formation in pathogenic bacteria". Cell Rep. 4 (4): 697–708. doi:10.1016/j.celrep.2013.07.026. PMC 3770465
. PMID 23954787.
- ↑ Zimmer, Carl. "Watching Bacteria Evolve, With Predictable Results". Retrieved 2 February 2016.
- Breidenstein EB, de la Fuente-Núñez C, Hancock RE (August 2011). "Pseudomonas aeruginosa: all roads lead to resistance". Trends Microbiol. 19 (8): 419–26. doi:10.1016/j.tim.2011.04.005. PMID 21664819.
External links
- "Type strain of Pseudomonas aeruginosa". BacDive — the Bacterial Diversity Metadatabase.
- "Pseudomonas aeruginosa". NCBI Taxonomy Browser. 287.