Cardiolipin
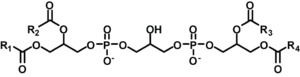
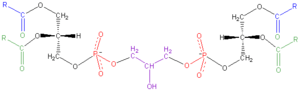
Cardiolipin (IUPAC name "1,3-bis(sn-3’-phosphatidyl)-sn-glycerol") is an important component of the inner mitochondrial membrane, where it constitutes about 20% of the total lipid composition. It can also be found in the membranes of most bacteria. The name ‘cardiolipin’ is derived from the fact that it was first found in animal hearts. It was first isolated from beef heart in the early 1940s.[1] In mammalian cells, but also in plant cells,[2][3] cardiolipin (CL) is found almost exclusively in the inner mitochondrial membrane where it is essential for the optimal function of numerous enzymes that are involved in mitochondrial energy metabolism.
Structure
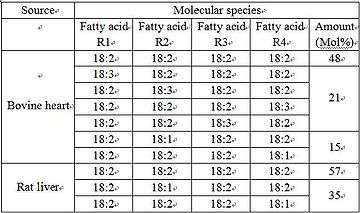
Cardiolipin (CL) is a kind of diphosphatidylglycerol lipid. Two phosphatidic acid moieties connect with a glycerol backbone in the center to form a dimeric structure. So it has four alkyl groups and potentially carries two negative charges. As there are four distinct alkyl chains in cardiolipin, the potential for complexity of this molecule species is enormous. However, in most animal tissues, cardiolipin contains 18-carbon fatty alkyl chains with 2 unsaturated bonds on each of them.[4] It has been proposed that the (18:2)4 acyl chain configuration is an important structural requirement for the high affinity of CL to inner membrane proteins in mammalian mitochondria.[5] However, studies with isolated enzyme preparations indicate that its importance may vary depending on the protein examined.
Since there are two phosphates in the molecule, each of them can catch one proton. Although it has a symmetric structure, ionizing one phosphate happens at a very different levels of acidity than ionizing both: pK1 = 3 and pK2 > 7.5. So under normal physiological conditions (wherein pH is around 7), the molecule may carry only one negative charge. The hydroxyl groups (–OH and –O−) on phosphate would form a stable intramolecular hydrogen bond with the centered glycerol’s hydroxyl group, thus forming a bicyclic resonance structure. This structure traps one proton, which is quite helpful for oxidative phosphorylation.
As the head group forms such compact bicycle structure, the head group area is quite small relative to the big tail region consisting of 4 acyl chains. Based on this special structure, the fluorescent mitochondrial indicator, nonyl acridine orange (NAO) was introduced in 1982,[6] and was later found to target mitochondria by binding to CL. NAO has a very large head and small tail structure which can compensate with cardiolipin’s small head large tail structure, and arrange in a highly ordered way.[7] Several studies were published utilizing NAO both as a quantitative mitochondrial indicator and an indicator of CL content in mitochondria. However, NAO is influenced by membrane potential and/or the spatial arrangement of CL,[8][9][10] so it's not proper to use NAO for CL or mitochondria quantitative studies of intact respiring mitochondria. But NAO still represents a simple method of assessing CL content. Cardiolipin has a molecular weight of 1466.0585 g/mol.
-
Cardiolipin bicyclic structure
-
Structure of NAO
-
NAO & CL arranged in a highly ordered way
Metabolism and catabolism

Metabolism
Eukaryotic pathway
In eukaryotes such as yeasts, plants and animals, the synthesis processes are believed to happen in mitochondria. The first step is the acylation of glycerol-3-phosphate by a glycerol-3-phosphate acyltransferase. Then acylglycerol-3-phosphate can be acylated to form a phosphatidic acid (PA). With the help of the enzyme CDP-DAG synthase (phosphatidate cytidylyltransferase), PA is converted into cytidinediphosphate-diacylglycerol (CDP-DAG). The following step is conversion of CDP-DAG to phosphatidylglycerol phosphate (PGP) by the enzyme PGP synthase, followed by dephosphorylation by PTPMT1 [11] to form PG. Finally, a molecule of CDP-DAG is bound to PG to form one molecule of cardiolipin, catalyzed by the mitochondria-localized enzyme cardiolipin synthase (CLS).[2][3][12]
Prokaryotic pathway
In prokaryotes such as bacteria, diphosphatidylglycerol synthase catalyses a transfer of the phosphatidyl moiety of one phosphatidylglycerol to the free 3'-hydroxyl group of another, with the elimination of one molecule of glycerol, via the action of an enzyme related to phospholipase D. The enzyme can operate in reverse under some physiological conditions to remove cardiolipin.
Catabolism
Catabolism of cardiolipin may happen by the catalysis of phospholipase A2 (PLA) to remove fatty acyl groups. Phospholipase D (PLD) in the mitochondrion hydrolyses cardiolipin to phosphatidic acid.[13]
Functions
Regulates aggregate structures
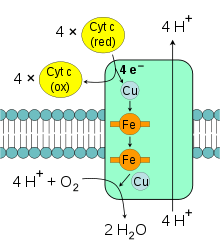
Because of cardiolipin’s unique structure, a change in pH and the presence of divalent cations can induce a structural change. CL shows a great variety of forms of aggregates. It is found that in the presence of Ca2+ or other divalent cations, CL can be induced to have a lamellar-to-hexagonal (La-HII) phase transition. And it is believed to have a close connection with membrane fusion.[14]
Helps to build quaternary structure
The enzyme cytochrome c oxidase or Complex IV is a large transmembrane protein complex found in bacteria and the mitochondrion. It is the last enzyme in the respiratory electron transport chain of mitochondria (or bacteria) located in the mitochondrial (or bacterial) membrane. It receives an electron from each of four cytochrome c molecules, and transfers them to one oxygen molecule, converting molecular oxygen to two molecules of water. Complex IV has been shown to require two associated CL molecules in order to maintain its full enzymatic function. Cytochrome bc1(Complex III) also needs cardiolipin to maintain its quaternary structure and to maintains its functional role.[15] Complex V of the oxidative phosphorylation machinery also displays high binding affinity for CL, binding four molecules of CL per molecule of complex V.[16]

Triggers apoptosis
Cardiolipin distribution to the outer mitochondrial membrane would lead to apoptosis of the cells, as evidenced by cytochrome c (cyt c) release, Caspase-8 activation, MOMP induction and NLRP3 inflammasome activation.[17] During apoptosis, cyt c is released from the intermembrane spaces of mitochondria into the cytosol. Cyt c can then bind to the IP3 receptor on ER, stimulating calcium release, which then reacts back to cause the release of cyt c. When the calcium concentration reaches a toxic level, this causes cell death. Cytochrome c is thought to play a role in apoptosis via the release of apoptotic factors from the mitochondria.[18] A cardiolipin-specific oxygenase produces CL hydroperoxides which can result in the conformation change of the lipid. The oxidized CL transfers from the inner membrane to the outer membrane, and then helps to form a permeable pore which releases cyt c.
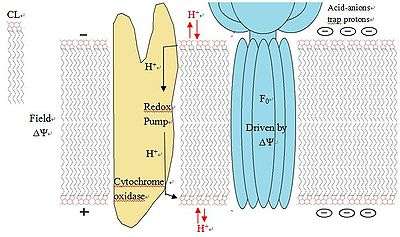
Serves as proton trap for oxidative phosphorylation
During the oxidative phosphorylation process catalyzed by Complex IV, large quantities of protons are transferred from one side of the membrane to another side causing a large pH change. CL is suggested to function as a proton trap within the mitochondrial membranes, thereby strictly localizing the proton pool and minimizing the changes in pH in the mitochondrial intermembrane space.
This function is due to CL’s unique structure. As stated above, CL can trap a proton within the bicyclic structure while carrying a negative charge. Thus, this bicyclic structure can serve as an electron buffer pool to release or absorb protons to maintain the pH near the membranes.[7]
Other functions
- Cholesterol translocation from outer to the inner membrane of mitochondrial
- Activates mitochondrial cholesterol side-chain cleavage
- Import protein into mitochondrial matrix
- Anticoagulant function
Clinical significance
Barth syndrome
Barth syndrome is a rare genetic disorder that was recognised in the 1970s to cause infantile death. It has a mutation in the gene coding for tafazzin, an enzyme involved in the biosynthesis of cardiolipin. Tafazzin is an indispensable enzyme to synthesize cardiolipin in eukaryotes involved in the remodeling of CL acyl chains by transferring linoleic acid from PC to monolysocardiolipin.[19] Mutation of tafazzin would cause insufficient cardiolipin remodeling and affect ATP production. Females heterozygous for the trait are unaffected. Sufferers of this condition have mitochondria that are abnormal, and they cannot sustain adequate production of ATP. Cardiomyopathy and general weakness is common to these patients.
Parkinson's disease and Alzheimer’s disease
Oxidative stress and lipid peroxidation are believed to be contributing factors leading to neuronal loss and mitochondrial dysfunction in the substantia nigra in Parkinson's disease, and may play an early role in the pathogenesis of Alzheimer's disease.[20][21] It is reported that CL content in the brain decreases with aging,[22] and a recent study on rat brain shows it results from lipid peroxidation in mitochondria exposed to free radical stress. Another study shows that the CL biosynthesis pathway may be selectively impaired, causing 20% reduction and composition change of the CL content.[23] It’s also associated with a 15% reduction in linked complex I/III activity of the electron transport chain, which is thought to be a critical factor in the development of Parkinson's disease.[24]
Nonalcoholic fatty liver disease and heart failure
Recently, it is reported that in non-alcoholic fatty liver disease[25] and heart failure[26], decreased CL levels and change in acyl chain composition are also observed in the mitochondrial dysfunction. However, the role of CL in aging and ischemia/reperfusion is still controversial.
Tangier disease
Tangier disease is also linked to CL abnormalities. Tangier disease is characterized by very low blood plasma levels of High-Density Lipoprotein cholesterol ("good cholesterol"), accumulation of cholesteryl esters in tissues and an increased risk for developing cardiovascular disease.[27] Unlike Barth syndrome, Tangier disease is mainly caused by abnormal enhanced production of CL. Studies show that there are three to fivefold increase of CL level in Tangier disease.[28] Because increased CL levels would enhance cholesterol oxidation, and then the formation of oxysterols would consequently increase cholesterol efflux. This process could function as an escape mechanism to remove excess cholesterol from the cell.
Diabetes
Heart disease hits people with diabetes twice as often as people without diabetes. In those with diabetes, cardiovascular complications occur at an earlier age and often result in premature death, making heart disease the major killer of diabetic people. Cardiolipin has recently been found to be deficient in the heart at the earliest stages of diabetes, possibly due to a lipid-digesting enzyme that becomes more active in diabetic heart muscle.[29]
Syphilis
Cardiolipin from a cow heart is used as an antigen in the Wassermann test for syphilis. Anti-cardiolipin antibodies can also be increased in numerous other conditions, including systemic lupus erythematosus, malaria and tuberculosis, so this test is not specific.
HIV-1
Human immunodeficiency virus-1 (HIV-1) has infected more than 60 million people worldwide. HIV-1 envelope glycoprotein contains at least four sites for neutralizing antibodies. Among these sites, the membrane-proximal region (MPR) is particularly attractive as an antibody target because it facilitates viral entry into T cells and is highly conserved among viral strains.[30] However, it is found that two antibodies directed against 2F5, 4E10 in MPR react with self-antigens, including cardiolipin. Thus, it’s difficult for such antibodies to be elicited by vaccination.[31]
Cancer
It was first proposed by Otto Heinrich Warburg that cancer originated from irreversible injury to mitochondrial respiration, but the structural basis for this injury has remained elusive. Since cardiolipin is an important phospholipid found almost exclusively in the inner mitochondrial membrane and very essential in maintaining mitochondrial function, it is suggested that abnormalities in CL can impair mitochondrial function and bioenergetics. A study[32] published in 2008 on mouse brain tumors supporting Warburg’s cancer theory shows major abnormalities in CL content or composition in all tumors.
Antiphospholipid syndrome
Patients with anti-cardiolipin antibodies (Antiphospholipid syndrome) can have recurrent thrombotic events even early in their mid- to late-teen years. These events can occur in vessels in which thrombosis may be relatively uncommon, such as the hepatic or renal veins. These antibodies are usually picked up in young women with recurrent spontaneous abortions. In anti-cardiolipin-mediated autoimmune disease, there is a dependency on the apolipoprotein H for recognition.[33]
Additional Anti-cardiolipin diseases
Bartonella Infection
Bartonellosis is a serious chronic bacterial infection shared by both cats and humans. Spinella found that one patient with bartonella henselae also had anti-cardiolipin antibodies, suggesting that bartonella may trigger their production.[34]
Chronic Fatigue syndrome
Chronic Fatigue Syndrome is debilitating illness of unknown cause that often follows an acute viral infection. According to one research study, 95% of CFS patients have anti-cardiolipin antibodies.[35]
See also
References
- ↑ Pangborn M. (1942). "Isolation and purification of a serologically active phospholipid from beef heart". J. Biol. Chem. 143: 247–256.
- 1 2 M. Nowicki; M. Frentzen (2005). "Cardiolipin synthase of Arabidopsis thaliana". FEBS Letters. 579 (10): 2161–2165. doi:10.1016/j.febslet.2005.03.007. PMID 15811335.
- 1 2 M. Nowicki (2006). "Characterization of the Cardiolipin Synthase from Arabidopsis thaliana". Ph.D. thesis, RWTH-Aachen University.
- ↑ Michael SCHLAME; Stuart BRODY; Karl Y. HOSTETLER (March 1993). "Mitochondrial cardiolipin in diverse eukaryotes". European Journal of Biochemistry. 212 (3): 727–733. doi:10.1111/j.1432-1033.1993.tb17711.x.
- ↑ Schlame M, Horvath L, Vigh L (1994). "Relationship between lipid saturation and lipid-protein interaction in liver mitochondria modified by catalytic hydrogenation with reference to cardiolipin molecular species". Biochem. J. 265 (1): 79–85. PMC 1136616
. PMID 2154183.
- ↑ Erbrich U, Naujok A, Petschel K, Zimmermann HW (1982). "The fluorescent staining of mitochondria in living HeLa- and LM-cells with new acridine dyes". Histochemistry. 74 (1): 1–7. PMID 7085344.
- 1 2 Thomas H. Haines; Norbert A. Dencher (2002). "Cardiolipin: a proton trap for oxidative phosphorylation". FEBS Lett. 528 (1–3): 35–39. doi:10.1016/S0014-5793(02)03292-1. PMID 12297275.
- ↑ M Garciafernandez; D Ceccarelli; U Muscatello (2004). "Use of the fluorescent dye 10-N-nonyl acridine orange in quantitative and location assays of cardiolipin: a study on different experimental models". Analytical Biochemistry. 328 (2): 174–180. doi:10.1016/j.ab.2004.01.020. PMID 15113694.
- ↑ Jacobson J, Duchen MR, Heales SJ (2002). "Intracellular distribution of the fluorescent dye nonyl acridine orange responds to the mitochondrial membrane potential: implications for assays of cardiolipin and mitochondrial". Mass. J Neurochem. 82 (2): 224–233. doi:10.1046/j.1471-4159.2002.00945.x.
- ↑ Keij JF, Bell-Prince C, Steinkamp JA (2000). "Staining of mitochondrial membranes with 10-nonyl acridine orange, MitoFluor Green, and Mito-Tracker Green is affected by mitochondrial membrane potential altering drugs". Cytometry. 39 (3): 203–210. doi:10.1002/(SICI)1097-0320(20000301)39:3<203::AID-CYTO5>3.0.CO;2-Z. PMID 10685077.
- ↑ Zhang, J; Dixon JE (8 June 2011). "Mitochondrial phosphatase PTPMT1 is essential for cardiolipin biosynthesis". Cell Metab. 13 (6): 690–700. doi:10.1016/j.cmet.2011.04.007. PMID 21641550.
- ↑ R. H. Houtkooper; F. M. Vaz (2008). "Cardiolipin, the heart of mitochondrial metabolism". Cell. Mol. Life Sci. 65 (16): 2493–2506. doi:10.1007/s00018-008-8030-5. PMID 18425414.
- ↑ Gregor Cevc. Phospholipids handbook. p. 783. ISBN 0-8247-9050-2.
- ↑ Antonio Ortiz; J. Antoinette Killian; Arie J. Verkleij; Jan Wilschut (1999). "Membrane fusion and the lamellar-to-inverted-hexagonal phase transition in cardiolipin vesicle systems induced by divalent cations". Biophysical Journal. 77 (4): 2003–2014. doi:10.1016/S0006-3495(99)77041-4. PMC 1300481
. PMID 10512820.
- ↑ Baltazar Gomez Jr.; Neal C. Robinson (1999). "Phospholipase Digestion of Bound Cardiolipin Reversibly Inactivates Bovine Cytochrome bc1". Biochemistry. 38 (28): 9031–9038. doi:10.1021/bi990603r. PMID 10413476.
- ↑ Eble KS, Coleman WB, Hantgan RR, Cunningham CC (1990). "Tightly associated cardiolipin in the bovine heart mitochondrial ATP synthase as analyzed by 31P nuclear magnetic resonance spectroscopy". J. Biol. Chem. 265 (32): 19434–19440. PMID 2147180.
- ↑ Paradies, G; Petrosillo, G; Paradies, V; Ruggiero, FM (2009). "Role of cardiolipin peroxidation and Ca2+ in mitochondrial dysfunction and disease". Cell Calcium. 45: 643–650. doi:10.1016/j.ceca.2009.03.012.
- ↑ Natalia A. Belikova; et al. (2006). "Peroxidase Activity and Structural Transitions of Cytochrome c Bound to Cardiolipin-Containing Membranes". Biochemistry. 45 (15): 4998–5009. doi:10.1021/bi0525573. PMC 2527545
. PMID 16605268.
- ↑ Xu Y.; Malhotra A.; Ren M.; Schlame M. (2006). "The enzymatic function of tafazzin". J. Biol. Chem. 281 (51): 39217–39224. doi:10.1074/jbc.M606100200. PMID 17082194.
- ↑ Beal MF. (2003). "Mitochondria, oxidative damage, and inflammation in Parkinson's disease". Ann NY Acad Sci. 991: 120–131. doi:10.1111/j.1749-6632.2003.tb07470.x. PMID 12846981.
- ↑ Jenner P. (1991). "Oxidative stress as a cause of Parkinson's disease". Acta Neurol Scand Suppl. 136: 6–15. doi:10.1002/ana.10483. PMID 12666096.
- ↑ Ruggiero FM, Cafagna F, Petruzzella V, Gadaleta MN, Quagliariello E (1991). "Lipid composition in synaptic and nonsynaptic mitochondria from rat brains and effect of aging". J Neurochem. 59 (2): 487–491. doi:10.1111/j.1471-4159.1992.tb09396.x. PMID 1629722.
- ↑ Ellis CE, Murphy EJ, Mitchell DC, Golovko MY, Scaglia F, Barcelo-Coblijn GC, Nussbaum RL (2005). "Mitochondrial Lipid Abnormality and Electron Transport Chain Impairment in Mice Lacking α-Synuclein". Mol Cell Biol. 25 (22): 10190–10201. doi:10.1128/MCB.25.22.10190-10201.2005. PMC 1280279
. PMID 16260631.
- ↑ Dawson TM, Dawson VL (2003). "Molecular pathways of neurodegeneration in Parkinson's disease". Science. 302 (5646): 819–822. doi:10.1126/science.1087753. PMID 14593166.
- ↑ Petrosillo G.; Portincasa P.; Grattagliano I.; Casanova G.; Matera M.; Ruggiero F. M.; Ferri D.; Paradies G. (2007). "Mitochondrial dysfunction in rat with nonalcoholic fatty liver: involvement of complex I, reactive oxygen species and cardiolipin". Biochim. Biophys. Acta. 1767 (10): 1260–1267. doi:10.1016/j.bbabio.2007.07.011. PMID 17900521.
- ↑ Sparagna G. C.; Chicco A. J.; Murphy R. C.; Bristow M. R.; Johnson C. A.; Rees M. L.; Maxey M. L.; McCune S. A.; Moore R. L. (2007). "Loss of cardiac tetralinoleoyl cardiolipin in human and experimental heart failure". J. Lipid Res. 48 (7): 1559–1570. doi:10.1194/jlr.M600551-JLR200. PMID 17426348.
- ↑ Oram J. F. (2000). "Tangier disease and ABCA1". Biochim. Biophys. Acta. 1529 (1–3): 321–330. doi:10.1016/S1388-1981(00)00157-8. PMID 11111099.
- ↑ Fobker M.; Voss R.; Reinecke H.; Crone C.; Assmann G.; Walter M. (2001). "Accumulation of cardiolipin and lysocardiolipin in fibroblasts from Tangier disease subjects". FEBS Lett. 500 (3): 157–162. doi:10.1016/S0014-5793(01)02578-9. PMID 11445077.
- ↑ Xianlin Han; et al. (2007). "Alterations in Myocardial Cardiolipin Content and Composition Occur at the Very Earliest Stages of Diabetes: A Shotgun Lipidomics Study". Biochemistry. 46 (21): 6417–6428. doi:10.1021/bi7004015. PMC 2139909
. PMID 17487985.
- ↑ Gary J. Nabel (2005). "Immunology: Close to the Edge: Neutralizing the HIV-1 Envelope". Science. 308 (5730): 1878–1879. doi:10.1126/science.1114854. PMID 15976295.
- ↑ J. M. Binley; et al. (2004). "Comprehensive Cross-Clade Neutralization Analysis of a Panel of Anti-Human Immunodeficiency Virus Type 1 Monoclonal Antibodies". J. Virol. 78 (23): 13232–13252. doi:10.1128/JVI.78.23.13232-13252.2004. PMC 524984
. PMID 15542675.
- ↑ Michael A. Kiebish; et al. (2008). "Cardiolipin and electron transport chain abnormalities in mouse brain tumor mitochondria: lipidomic evidence supporting the Warburg theory of cancer". Journal of Lipid Research. 49 (12): 2545–2556. doi:10.1194/jlr.M800319-JLR200. PMC 2582368
. PMID 18703489.
- ↑ McNeil HP, Simpson RJ, Chesterman CN, Krilis SA (1990). "Anti-phospholipid antibodies are directed against a complex antigen that includes a lipid-binding inhibitor of coagulation: beta 2-glycoprotein I (apolipoprotein H)". Proc. Natl. Acad. Sci. U.S.A. 87 (11): 4120–4. doi:10.1073/pnas.87.11.4120. PMC 54059
. PMID 2349221.
- ↑ Spinella A, Lumetti F, Sandri G, Cestelli V, Mascia MT. Beyond cat scratch disease: a case report of bartonella infection mimicking vasculitic disorder. Case Rep Infect Dis. 2012;2012:354625.
- ↑ Hokama Y, Campora CE, Hara C, Kuribayashi T, Le Huynh D, Yabusaki K. Anticardiolipin antibodies in the sera of patients with diagnosed chronic fatigue syndrome. J Clin Lab Anal. 2009;23(4):210-2.
External links
- Cardiolipin at the US National Library of Medicine Medical Subject Headings (MeSH)
- Cardiolipin (Diphosphatidylglycerol)
- Some Methodological Developments in Phospholipid Chemistry & Physico-Chemical Studies of Calcium-ion Induced Changes in Cardiolipin Vesicles