Milky Way
The Milky Way's Galactic Center in the night sky above Paranal Observatory (the laser creates a guide-star for the telescope). | |
Observation data | |
---|---|
Type | Sb, Sbc, or SB(rs)bc[1][2] (barred spiral galaxy) |
Diameter | 100–180 kly (31–55 kpc)[3] |
Thickness of thin stellar disk | ≈2 kly (0.6 kpc)[4][5] |
Number of stars | 100–400 billion (2.5×1011 ±1.5×1011)[6][7][8] |
Oldest known star | ≥13.7 Gyr[9] |
Mass | 0.8–1.5×1012 M☉[10][11][12] |
Angular momentum | ≈×1067 J s 1[13] |
Sun's distance to Galactic Center | 27.2 ± 1.1 kly (8.34 ± 0.34 kpc)[14] |
Sun's Galactic rotation period | 240 Myr[15] |
Spiral pattern rotation period | 220–360 Myr[16] |
Bar pattern rotation period | 100–120 Myr[16] |
Speed relative to CMB rest frame | 552 ± 6 km/s[17] |
Escape velocity at Sun's position | 550 km/s[12] |
Dark matter density at Sun's position |
0.0088+0.0024 -0.0018 M☉pc-3 or 0.35+0.08 -0.07 GeV cm-3[12] |
See also: Galaxy, List of galaxies |
The Milky Way is the galaxy that contains our Solar System.[18][19][20][nb 1] Its name "milky" is derived from its appearance as a dim glowing band arching across the night sky whose individual stars cannot be distinguished by the naked eye. The term "Milky Way" is a translation of the Latin via lactea, from the Greek γαλαξίας κύκλος (galaxías kýklos, "milky circle").[21][22][23] From Earth, the Milky Way appears as a band because its disk-shaped structure is viewed from within. Galileo Galilei first resolved the band of light into individual stars with his telescope in 1610. Until the early 1920s, most astronomers thought that the Milky Way contained all the stars in the Universe. Following the 1920 Great Debate between the astronomers Harlow Shapley and Heber Curtis,[24] observations by Edwin Hubble showed that the Milky Way is just one of many galaxies. Subsequent sensitive observations such as the Hubble telescope's Ultra Deep Field revealed a myriad of faint galaxies, which led to an estimate that the observable universe contained about 200 billion galaxies.[25][26] A 2016 study estimated that the observable universe contained ten times that number or 2 trillion galaxies.[27]
The Milky Way is a barred spiral galaxy that has a diameter usually considered to be about 100,000–120,000 light-years[28] but may be 150,000–180,000 light-years.[29] The Milky Way is estimated to contain 100–400 billion stars.[30][31] There are likely at least 100 billion planets in the Milky Way.[32][33] The Solar System is located within the disk, about 27,000 light-years from the Galactic Center, on the inner edge of one of the spiral-shaped concentrations of gas and dust called the Orion Arm. The stars in the inner ≈10,000 light-years form a bulge and one or more bars that radiate from the bulge. The very center is marked by an intense radio source, named Sagittarius A*, which is likely to be a supermassive black hole.
Stars and gases at a wide range of distances from the Galactic Center orbit at approximately 220 kilometers per second. The constant rotation speed contradicts the laws of Keplerian dynamics and suggests that much of the mass of the Milky Way does not emit or absorb electromagnetic radiation. This mass has been termed "dark matter".[34] The rotational period is about 240 million years at the position of the Sun.[15] The Milky Way as a whole is moving at a velocity of approximately 600 km per second with respect to extragalactic frames of reference. The oldest stars in the Milky Way are nearly as old as the Universe itself and thus likely formed shortly after the Dark Ages of the Big Bang.[9]
The Milky Way has several satellite galaxies and is part of the Local Group of galaxies, which is a component of the Virgo Supercluster, which is itself a component of the Laniakea Supercluster.[35][36]
Appearance
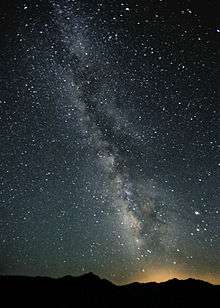
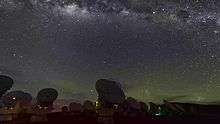
The "Milky Way" can be seen as a hazy band of white light some 30 degrees wide arcing across the sky.[37] Although all the individual naked-eye stars in the entire sky are part of the Milky Way,[38] the light in this band originates from the accumulation of unresolved stars and other material located in the direction of the galactic plane. Dark regions within the band, such as the Great Rift and the Coalsack, are areas where light from distant stars is blocked by interstellar dust. The area of the sky obscured by the Milky Way is called the Zone of Avoidance.
The Milky Way has a relatively low surface brightness. Its visibility can be greatly reduced by background light such as light pollution or stray light from the Moon. The sky needs to be darker than about 20.2 magnitude per square arcsecond in order for the Milky Way to be seen.[39] It should be visible when the limiting magnitude is approximately +5.1 or better and shows a great deal of detail at +6.1.[40] This makes the Milky Way difficult to see from any brightly lit urban or suburban location, but very prominent when viewed from a rural area when the Moon is below the horizon.[nb 2] The new world atlas of artificial night sky brightness shows that more than one third of Earth population cannot see the Milky Way from their homes due to light pollution.[41]
As viewed from Earth, the visible region of the Milky Way's Galactic plane occupies an area of the sky that includes 30 constellations.[42] The center of the Galaxy lies in the direction of the constellation Sagittarius; it is here that the Milky Way is brightest. From Sagittarius, the hazy band of white light appears to pass around to the Galactic anticenter in Auriga. The band then continues the rest of the way around the sky, back to Sagittarius. The band divides the night sky into two roughly equal hemispheres.
The Galactic plane is inclined by about 60 degrees to the ecliptic (the plane of Earth's orbit). Relative to the celestial equator, it passes as far north as the constellation of Cassiopeia and as far south as the constellation of Crux, indicating the high inclination of Earth’s equatorial plane and the plane of the ecliptic, relative to the Galactic plane. The north Galactic pole is situated at right ascension 12h 49m, declination +27.4° (B1950) near β Comae Berenices, and the south Galactic pole is near α Sculptoris. Because of this high inclination, depending on the time of night and year, the arc of Milky Way may appear relatively low or relatively high in the sky. For observers from approximately 65 degrees north to 65 degrees south on Earth's surface, the Milky Way passes directly overhead twice a day.

Size and mass
The Milky Way is the second-largest galaxy in the Local Group, with its stellar disk approximately 100,000 ly (30 kpc) in diameter, and, on average, approximately 1,000 ly (0.3 kpc) thick.[4][5] As a guide to the relative physical scale of the Milky Way, if the Solar System out to Neptune were the size of a US quarter (24.3 mm (0.955 in)), the Milky Way would be approximately the size of the continental United States.[44] A ring-like filament of stars wrapping around the Milky Way may actually belong to the Milky Way itself, rippling above and below the relatively flat galactic plane.[29] If so, that would mean a diameter of 150,000–180,000 light-years (46–55 kpc).[45]
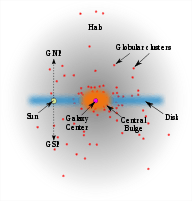
Estimates of the mass of the Milky Way vary, depending upon the method and data used. At the low end of the estimate range, the mass of the Milky Way is 5.8×1011 solar masses (M☉), somewhat less than that of the Andromeda Galaxy.[46][47][48] Measurements using the Very Long Baseline Array in 2009 found velocities as large as 254 km/s (570,000 mph) for stars at the outer edge of the Milky Way.[49] Because the orbital velocity depends on the total mass inside the orbital radius, this suggests that the Milky Way is more massive, roughly equaling the mass of Andromeda Galaxy at 7×1011 M☉ within 160,000 ly (49 kpc) of its center.[50] In 2010, a measurement of the radial velocity of halo stars found that the mass enclosed within 80 kiloparsecs is 7×1011 M☉.[51] According to a study published in 2014, the mass of the entire Milky Way is estimated to be 8.5×1011 M☉,[52] which is about half the mass of the Andromeda Galaxy.[52]
Most of the mass of the Milky Way appears to be dark matter, an unknown and invisible form of matter that interacts gravitationally with ordinary matter. A dark matter halo is spread out relatively uniformly to a distance beyond one hundred kiloparsecs from the Galactic Center. Mathematical models of the Milky Way suggest that its total mass is 1–1.5×1012 M☉.[10] More-recent studies indicate a mass as large as 4.5×1012 M☉ [53] and as small as 0.8×1012 M☉.[54]
The total mass of all the stars in the Milky Way is estimated to be between 4.6×1010 M☉[55] and 6.43×1010 M☉.[10] In addition to the stars, there is also interstellar gas, comprising 90% hydrogen and 10% helium by mass,[56] with two thirds of the hydrogen found in the form of atomic form and the remaining one third as molecular hydrogen.[57] The mass of this gas is equal to between 10%[57] and 15%[56] of the total mass of the galaxy's stars. Interstellar dust accounts for an additional 1% of the total mass of the gas.[56]
Contents
The Milky Way contains between 200 and 400 billion stars[58][59] and at least 100 billion planets.[60] The exact figure depends on the number of very-low-mass stars, which are hard to detect, especially at distances of more than 300 ly (90 pc) from the Sun. As a comparison, the neighboring Andromeda Galaxy contains an estimated one trillion (1012) stars.[61] Filling the space between the stars is a disk of gas and dust called the interstellar medium. This disk has at least a comparable extent in radius to the stars,[62] whereas the thickness of the gas layer ranges from hundreds of light years for the colder gas to thousands of light years for warmer gas.[63][64]
The disk of stars in the Milky Way does not have a sharp edge beyond which there are no stars. Rather, the concentration of stars decreases with distance from the center of the Milky Way. For reasons that are not understood, beyond a radius of roughly 40,000 ly (13 kpc) from the center, the number of stars per cubic parsec drops much faster with radius.[65] Surrounding the galactic disk is a spherical Galactic Halo of stars and globular clusters that extends further outward but is limited in size by the orbits of two Milky Way satellites, the Large and Small Magellanic Clouds, whose closest approach to the Galactic Center is about 180,000 ly (55 kpc).[66] At this distance or beyond, the orbits of most halo objects would be disrupted by the Magellanic Clouds. Hence, such objects would probably be ejected from the vicinity of the Milky Way. The integrated absolute visual magnitude of the Milky Way is estimated to be around −20.9.[67][68][lower-alpha 1]
Both gravitational microlensing and planetary transit observations indicate that there may be at least as many planets bound to stars as there are stars in the Milky Way,[32][69] and microlensing measurements indicate that there are more rogue planets not bound to host stars than there are stars.[70][71] The Milky Way contains at least one planet per star, resulting in 100–400 billion planets, according to a January 2013 study of the five-planet star system Kepler-32 with the Kepler space observatory.[33] A different January 2013 analysis of Kepler data estimated that at least 17 billion Earth-sized exoplanets reside in the Milky Way.[72] On November 4, 2013, astronomers reported, based on Kepler space mission data, that there could be as many as 40 billion Earth-sized planets orbiting in the habitable zones of Sun-like stars and red dwarfs within the Milky Way.[73][74][75] 11 billion of these estimated planets may be orbiting Sun-like stars.[76] The nearest such planet may be 12 light-years away, according to scientists.[73][74] Such Earth-sized planets may be more numerous than gas giants.[32] Besides exoplanets, "exocomets", comets beyond the Solar System, have also been detected and may be common in the Milky Way.[77]
-
360-degree panorama view of the Milky Way (an assembled mosaic of photographs) by ESO. The galactic centre is in the middle of the view, with galactic north up.
Structure
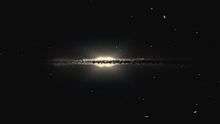
.jpg)

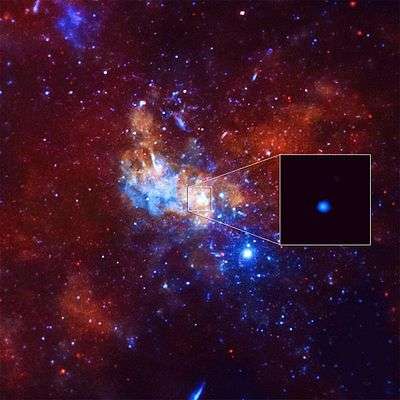
The Milky Way consists of a bar-shaped core region surrounded by a disk of gas, dust and stars. The gas, dust and stars are organized in roughly logarithmic spiral arm structures (see Spiral arms below). The mass distribution within the Milky Way closely resembles the type Sbc in the Hubble classification, which represents spiral galaxies with relatively loosely wound arms.[1] Astronomers first began to suspect that the Milky Way is a barred spiral galaxy, rather than an ordinary spiral galaxy, in the 1990s.[80] Their suspicions were confirmed by the Spitzer Space Telescope observations in 2005[81] that showed the Milky Way's central bar to be larger than previously thought.
Galactic quadrants
A galactic quadrant, or quadrant of the Milky Way, refers to one of four circular sectors in the division of the Milky Way. In actual astronomical practice, the delineation of the galactic quadrants is based upon the galactic coordinate system, which places the Sun as the origin of the mapping system.[82]
Quadrants are described using ordinals—for example, "1st galactic quadrant",[83] "second galactic quadrant",[84] or "third quadrant of the Milky Way".[85] Viewing from the north galactic pole with 0 degrees (°) as the ray that runs starting from the Sun and through the Galactic Center, the quadrants are as follows:
- 1st galactic quadrant – 0° ≤ longitude (ℓ) ≤ 90°[86]
- 2nd galactic quadrant – 90° ≤ ℓ ≤ 180°[84]
- 3rd galactic quadrant – 180° ≤ ℓ ≤ 270°[85]
- 4th galactic quadrant – 270° ≤ ℓ ≤ 360° (0°)[83]
Galactic Center
The Sun is 26,000–28,000 ly (8.0–8.6 kpc) from the Galactic Center. This value is estimated using geometric-based methods or by measuring selected astronomical objects that serve as standard candles, with different techniques yielding various values within this approximate range.[14][87][88][89][90] In the inner few kpc (around 10,000 light-years radius) is a dense concentration of mostly old stars in a roughly spheroidal shape called the bulge.[91] It has been proposed that the Milky Way lacks a bulge formed due to a collision and merger between previous galaxies and that instead has a pseudobulge formed by its central bar.[92]
The Galactic Center is marked by an intense radio source named Sagittarius A* (pronounced Sagittarius A-star). The motion of material around the center indicates that Sagittarius A* harbors a massive, compact object.[93] This concentration of mass is best explained as a supermassive black hole[nb 3][14][87] (SMBH) with an estimated mass of 4.1–4.5 million times the mass of the Sun.[87] The rate of accretion of the SMBH is consistent with an inactive galactic nucleus, being estimated at around ×10−5 1 M☉ y−1.[94] Observations indicate that there are SMBH located near the center of most normal galaxies.[95][96]
The nature of the Milky Way's bar is actively debated, with estimates for its half-length and orientation spanning from 1 to 5 kpc (3,000–16,000 ly) and 10–50 degrees relative to the line of sight from Earth to the Galactic Center.[89][90][97] Certain authors advocate that the Milky Way features two distinct bars, one nestled within the other.[98] However, RR Lyr variables do not trace a prominent Galactic bar.[90][99][100] The bar may be surrounded by a ring called the "5-kpc ring" that contains a large fraction of the molecular hydrogen present in the Milky Way, as well as most of the Milky Way's star-formation activity. Viewed from the Andromeda Galaxy, it would be the brightest feature of the Milky Way.[101] X-ray emission from the core is aligned with the massive stars surrounding the central bar[94] and the Galactic ridge.[102]
In 2010, two gigantic spherical bubbles of high energy emission were detected to the north and the south of the Milky Way core, using data of the Fermi Gamma-ray Space Telescope. The diameter of each of the bubbles is about 25,000 light-years (7.7 kpc); they stretch up to Grus and to Virgo on the night-sky of the southern hemisphere.[103][104] Subsequently, observations with the Parkes Telescope at radio frequencies identified polarized emission that is associated with the Fermi bubbles. These observations are best interpreted as a magnetized outflow driven by star formation in the central 640 ly (200 pc) of the Milky Way.[105]
Later, on January 5, 2015, NASA reported observing an X-ray flare 400 times brighter than usual, a record-breaker, from Sagittarius A*. The unusual event may have been caused by the breaking apart of an asteroid falling into the black hole or by the entanglement of magnetic field lines within gas flowing into Sagittarius A*, according to astronomers.[79]
Spiral arms
Outside the gravitational influence of the Galactic bars, astronomers generally organize the structure of the interstellar medium and stars in the disk of the Milky Way into four spiral arms.[106] Spiral arms typically contain a higher density of interstellar gas and dust than the Galactic average as well as a greater concentration of star formation, as traced by H II regions[107][108] and molecular clouds.[109]
The Milky Way's spiral structure is uncertain and there is currently no consensus on the nature of the Milky Way's spiral arms.[78] Perfect logarithmic spiral patterns only crudely describe features near the Sun,[108][110] because galaxies commonly have arms that branch, merge, twist unexpectedly, and feature a degree of irregularity.[90][110][111] The possible scenario of the Sun within a spur / Local arm[108] emphasizes that point and indicates that such features are probably not unique, and exist elsewhere in the Milky Way.[110]
As in most spiral galaxies, each spiral arm can be described as a logarithmic spiral. Estimates of the pitch angle of the arms range from about 7° to 25°.[62][112] There are thought to be four spiral arms that all start near the Milky Way's center. These are named as follows, with the positions of the arms shown in the image at right:

Color | Arm(s) |
---|---|
cyan | 3-kpc Arm (Near 3 kpc Arm and Far 3 kpc Arm) and Perseus Arm |
purple | Norma and Outer arm (Along with extension discovered in 2004[113]) |
green | Scutum–Centaurus Arm |
pink | Carina–Sagittarius Arm |
There are at least two smaller arms or spurs, including: | |
orange | Orion–Cygnus Arm (which contains the Sun and Solar System) |
Two spiral arms, the Scutum–Centaurus arm and the Carina–Sagittarius arm, have tangent points inside the Sun's orbit about the center of the Milky Way. If these arms contain an overdensity of stars compared to the average density of stars in the Galactic disk, it would be detectable by counting the stars near the tangent point. Two surveys of near-infrared light, which is sensitive primarily to red giants and not affected by dust extinction, detected the predicted overabundance in the Scutum–Centaurus arm but not in the Carina–Sagittarius arm: the Scutum-Centaurus Arm contains approximately 30% more red giants than would be expected in the absence of a spiral arm.[112][114] In 2008, Robert Benjamin of the University of Wisconsin–Whitewater used this observation to suggest that the Milky Way possesses only two major stellar arms: the Perseus arm and the Scutum–Centaurus arm. The rest of the arms contain excess gas but not excess old stars.[78] In December 2013, astronomers found that the distribution of young stars and star-forming regions matches the four-arm spiral description of the Milky Way.[115][116][117] Thus, the Milky Way appears to have two spiral arms as traced by old stars and four spiral arms as traced by gas and young stars. The explanation for this apparent discrepancy is unclear.[117]
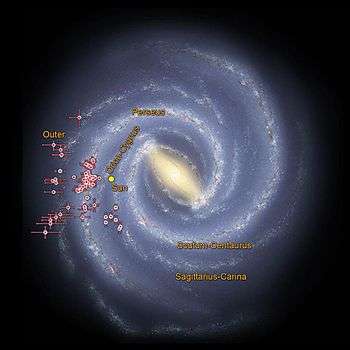
The Near 3 kpc Arm (also called Expanding 3 kpc Arm or simply 3 kpc Arm) was discovered in the 1950s by astronomer van Woerden and collaborators through 21-centimeter radio measurements of HI (atomic hydrogen).[118][119] It was found to be expanding away from the central bulge at more than 50 km/s. It is located in the fourth galactic quadrant at a distance of about 5.2 kpc from the Sun and 3.3 kpc from the Galactic Center. The Far 3 kpc Arm was discovered in 2008 by astronomer Tom Dame (Harvard-Smithsonian CfA). It is located in the first galactic quadrant at a distance of 3 kpc (about 10,000 ly) from the Galactic Center.[119][120]
A simulation published in 2011 suggested that the Milky Way may have obtained its spiral arm structure as a result of repeated collisions with the Sagittarius Dwarf Elliptical Galaxy.[121]
It has been suggested that the Milky Way contains two different spiral patterns: an inner one, formed by the Sagittarius arm, that rotates fast and an outer one, formed by the Carina and Perseus arms, whose rotation velocity is slower and whose arms are tightly wound. In this scenario, suggested by numerical simulations of the dynamics of the different spiral arms, the outer pattern would form an outer pseudoring[122] and the two patterns would be connected by the Cygnus arm.[123]
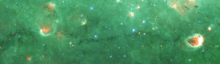
Outside of the major spiral arms is the Monoceros Ring (or Outer Ring), a ring of gas and stars torn from other galaxies billions of years ago. However, several members of the scientific community recently restated their position affirming the Monoceros structure is nothing more than an over-density produced by the flared and warped thick disk of the Milky Way.[124]
Halo
The Galactic disk is surrounded by a spheroidal halo of old stars and globular clusters, of which 90% lie within 100,000 light-years (30 kpc) of the Galactic Center.[125] However, a few globular clusters have been found farther, such as PAL 4 and AM1 at more than 200,000 light-years from the Galactic Center. About 40% of the Milky Way's clusters are on retrograde orbits, which means they move in the opposite direction from the Milky Way rotation.[126] The globular clusters can follow rosette orbits about the Milky Way, in contrast to the elliptical orbit of a planet around a star.[127]
Although the disk contains dust that obscures the view in some wavelengths, the halo component does not. Active star formation takes place in the disk (especially in the spiral arms, which represent areas of high density), but does not take place in the halo, as there is little gas cool enough to collapse into stars.[15] Open clusters are also located primarily in the disk.[128]
Discoveries in the early 21st century have added dimension to the knowledge of the Milky Way's structure. With the discovery that the disk of the Andromeda Galaxy (M31) extends much further than previously thought,[129] the possibility of the disk of the Milky Way extending further is apparent, and this is supported by evidence from the discovery of the Outer Arm extension of the Cygnus Arm[113][130] and of a similar extension of the Scutum-Centaurus Arm.[131] With the discovery of the Sagittarius Dwarf Elliptical Galaxy came the discovery of a ribbon of galactic debris as the polar orbit of the dwarf and its interaction with the Milky Way tears it apart. Similarly, with the discovery of the Canis Major Dwarf Galaxy, it was found that a ring of galactic debris from its interaction with the Milky Way encircles the Galactic disk.
On January 9, 2006, Mario Jurić and others of Princeton University announced that the Sloan Digital Sky Survey of the northern sky found a huge and diffuse structure (spread out across an area around 5,000 times the size of a full moon) within the Milky Way that does not seem to fit within current models. The collection of stars rises close to perpendicular to the plane of the spiral arms of the Milky Way. The proposed likely interpretation is that a dwarf galaxy is merging with the Milky Way. This galaxy is tentatively named the Virgo Stellar Stream and is found in the direction of Virgo about 30,000 light-years (9 kpc) away.[132]
Gaseous halo
In addition to the stellar halo, the Chandra X-ray Observatory, XMM-Newton, and Suzaku have provided evidence that there is a gaseous halo with a large amount of hot gas. The halo extends for hundreds of thousand of light years, much further than the stellar halo and close to the distance of the Large and Small Magellanic Clouds. The mass of this hot halo is nearly equivalent to the mass of the Milky Way itself.[133][134][135] The temperature of this halo gas is between 1 and 2.5 million K (1.8 and 4.5 million oF).[136]
Observations of distant galaxies indicate that the Universe had about one-sixth as much baryonic (ordinary) matter as dark matter when it was just a few billion years old. However, only about half of those baryons are accounted for in the modern Universe based on observations of nearby galaxies like the Milky Way.[137] If the finding that the mass of the halo is comparable to the mass of the Milky Way is confirmed, it could be the identity of the missing baryons around the Milky Way.[137]
Sun’s location and neighborhood
.png)
The Sun is near the inner rim of the Orion Arm, within the Local Fluff of the Local Bubble, and in the Gould Belt, at a distance of 8.33 ± 0.35 kiloparsecs (27,200 ± 1,100 ly) from the Galactic Center.[14][87][138] The Sun is currently 5–30 parsecs (16–98 ly) from the central plane of the Galactic disk.[139] The distance between the local arm and the next arm out, the Perseus Arm, is about 2,000 parsecs (6,500 ly).[140] The Sun, and thus the Solar System, is located in the Milky Way's galactic habitable zone.
There are about 208 stars brighter than absolute magnitude 8.5 within a sphere with a radius of 15 parsecs (49 ly) from the Sun, giving a density of one star per 69 cubic parsec, or one star per 2,360 cubic light-year (from List of nearest bright stars). On the other hand, there are 64 known stars (of any magnitude, not counting 4 brown dwarfs) within 5 parsecs (16 ly) of the Sun, giving a density of about one star per 8.2 cubic parsec, or one per 284 cubic light-year (from List of nearest stars). This illustrates the fact that there are far more faint stars than bright stars: in the entire sky, there are about 500 stars brighter than apparent magnitude 4 but 15.5 million stars brighter than apparent magnitude 14.[143]
The apex of the Sun's way, or the solar apex, is the direction that the Sun travels through space in the Milky Way. The general direction of the Sun's Galactic motion is towards the star Vega near the constellation of Hercules, at an angle of roughly 60 sky degrees to the direction of the Galactic Center. The Sun's orbit about the Milky Way is expected to be roughly elliptical with the addition of perturbations due to the Galactic spiral arms and non-uniform mass distributions. In addition, the Sun oscillates up and down relative to the Galactic plane approximately 2.7 times per orbit. This is very similar to how a simple harmonic oscillator works with no drag force (damping) term. These oscillations were until recently thought to coincide with mass lifeform extinction periods on Earth.[144] However, a reanalysis of the effects of the Sun's transit through the spiral structure based on CO data has failed to find a correlation.[145]
It takes the Solar System about 240 million years to complete one orbit of the Milky Way (a galactic year),[15] so the Sun is thought to have completed 18–20 orbits during its lifetime and 1/1250 of a revolution since the origin of humans. The orbital speed of the Solar System about the center of the Milky Way is approximately 220 km/s (490,000 mph) or 0.073% of the speed of light. The Sun moves through the heliosphere at 84,000 km/h (52,000 mph). At this speed, it takes around 1,400 years for the Solar System to travel a distance of 1 light-year, or 8 days to travel 1 AU (astronomical unit).[146] The Solar System is headed in the direction of the zodiacal constellation Scorpius, which follows the ecliptic.[147]
Galactic rotation
The stars and gas in the Milky Way rotate about its center differentially, meaning that the rotation period varies with location. As is typical for spiral galaxies, the orbital speed of most stars in the Milky Way does not depend strongly on their distance from the center. Away from the central bulge or outer rim, the typical stellar orbital speed is between 210 and 240 km/s (470,000 and 540,000 mph).[148] Hence the orbital period of the typical star is directly proportional only to the length of the path traveled. This is unlike the situation within the Solar System, where two-body gravitational dynamics dominate and different orbits have significantly different velocities associated with them. The rotation curve (shown in the figure) describes this rotation. Toward the center of the Milky Way the orbit speeds are too low, whereas beyond 7 kpcs the speeds are too high to match what would be expected from the universal law of gravitation.
If the Milky Way contained only the mass observed in stars, gas, and other baryonic (ordinary) matter, the rotation speed would decrease with distance from the center. However, the observed curve is relatively flat, indicating that there is additional mass that cannot be detected directly with electromagnetic radiation. This inconsistency is attributed to dark matter.[34] The rotation curve of the Milky Way agrees with the universal rotation curve of spiral galaxies, the best evidence for the existence of dark matter in galaxies. Alternatively, a minority of astronomers propose that a modification of the law of gravity may explain the observed rotation curve.[149]
Formation
The Milky Way began as one or several small overdensities in the mass distribution in the Universe shortly after the Big Bang. Some of these overdensities were the seeds of globular clusters in which the oldest remaining stars in what is now the Milky Way formed. These stars and clusters now comprise the stellar halo of the Milky Way. Within a few billion years of the birth of the first stars, the mass of the Milky Way was large enough so that it was spinning relatively quickly. Due to conservation of angular momentum, this led the gaseous interstellar medium to collapse from a roughly spheroidal shape to a disk. Therefore, later generations of stars formed in this spiral disk. Most younger stars, including the Sun, are observed to be in the disk.[150][151]
Since the first stars began to form, the Milky Way has grown through both galaxy mergers (particularly early in the Milky Way's growth) and accretion of gas directly from the Galactic halo.[151] The Milky Way is currently accreting material from two of its nearest satellite galaxies, the Large and Small Magellanic Clouds, through the Magellanic Stream. Direct accretion of gas is observed in high-velocity clouds like the Smith Cloud.[152][153] However, properties of the Milky Way such as stellar mass, angular momentum, and metallicity in its outermost regions suggest it has undergone no mergers with large galaxies in the last 10 billion years. This lack of recent major mergers is unusual among similar spiral galaxies; its neighbour the Andromeda Galaxy appears to have a more typical history shaped by more recent mergers with relatively large galaxies.[154][155]
According to recent studies, the Milky Way as well as the Andromeda Galaxy lie in what in the galaxy color–magnitude diagram is known as the "green valley", a region populated by galaxies in transition from the "blue cloud" (galaxies actively forming new stars) to the "red sequence" (galaxies that lack star formation). Star-formation activity in green valley galaxies is slowing as they run out of star-forming gas in the interstellar medium. In simulated galaxies with similar properties, star formation will typically have been extinguished within about five billion years from now, even accounting for the expected, short-term increase in the rate of star formation due to the collision between both the Milky Way and the Andromeda Galaxy.[156] In fact, measurements of other galaxies similar to the Milky Way suggest it is among the reddest and brightest spiral galaxies that are still forming new stars and it is just slightly bluer than the bluest red sequence galaxies.[157]
Age and cosmological history
.jpg)
Globular clusters are among the oldest objects in the Milky Way, which thus set a lower limit on the age of the Milky Way. The ages of individual stars in the Milky Way can be estimated by measuring the abundance of long-lived radioactive elements such as thorium-232 and uranium-238, then comparing the results to estimates of their original abundance, a technique called nucleocosmochronology. These yield values of about 12.5 ± 3 billion years for CS 31082-001[159] and 13.8 ± 4 billion years for BD +17° 3248.[160] Once a white dwarf is formed, it begins to undergo radiative cooling and the surface temperature steadily drops. By measuring the temperatures of the coolest of these white dwarfs and comparing them to their expected initial temperature, an age estimate can be made. With this technique, the age of the globular cluster M4 was estimated as 12.7 ± 0.7 billion years. Age estimates of the oldest of these clusters gives a best fit estimate of 12.6 billion years, and a 95% confidence upper limit of 16 billion years.[161]
Several individual stars have been found in the Milky Way's halo with measured ages very close to the 13.80-billion-year age of the Universe. In 2007, a star in the galactic halo, HE 1523-0901, was estimated to be about 13.2 billion years old. As the oldest known object in the Milky Way at that time, this measurement placed a lower limit on the age of the Milky Way.[162] This estimate was made using the UV-Visual Echelle Spectrograph of the Very Large Telescope to measure the relative strengths of spectral lines caused by the presence of thorium and other elements created by the R-process. The line strengths yield abundances of different elemental isotopes, from which an estimate of the age of the star can be derived using nucleocosmochronology.[162] Another star, HD 140283, is 14.5 ± 0.7 billion years old and thus formed at least 13.8 billion years ago.[9][163]
The age of stars in the galactic thin disk has also been estimated using nucleocosmochronology. Measurements of thin disk stars yield an estimate that the thin disk formed 8.8 ± 1.7 billion years ago. These measurements suggest there was a hiatus of almost 5 billion years between the formation of the galactic halo and the thin disk.[164] Recently Misha Hayward and his team at the Paris Observatory, through studying the chemical signatures of thousands of stars, have suggested that stellar formation may have dropped by an order of magnitude between 10 and 8 billion years ago. They suggest that this seems to have occurred at the time of disk formation, suggesting that the disc and barred structure by stirring up the gas made it too hot to form new stars at the same rate as before.[165]
Donald Lynden-Bell at Cambridge University in 1976[166] showed that the satellite galaxies surrounding the Milky way are not randomly distributed, but seemed to be the result of a break-up of some larger system producing a ring structure 500,000 light years in diameter and 50,000 light years wide. Close encounters between galaxies, like that expected in 4 billion years with the Andromeda Galaxy rips off huge tails of gas, which, over time can coalesce to form dwarf galaxies in a ring at right angles to the main disc. Pavel Kroupa,[167] and his doctoral student Pawlowski at the University of Bonn, studying the alignment of globular clusters and the whispy tails of the breakup of dwarf galaxies found they too formed part of such rings of matter. In 2013 Rodrigo Ibata, of the University of Strasbourg in France, has shown a similar ring structure exists around Andromeda Galaxy,[168] as a part of a coherent rotating structure, suggesting the Milky Way and Andromeda had interacted in the past. But this is impossible even taking account of the hidden dark matter haloes. A subtle change such as that suggested by the MOND model allows for an interaction between the two galaxies 7–11 billion years ago. Justin Koury of the University of Pennsylvania has suggested that if dark matter can involve a superfluid Bose-Einstein condensate it mimics the MOND model at certain states.[169]
Environment
.png)
.png)
The Milky Way and the Andromeda Galaxy are a binary system of giant spiral galaxies belonging to a group of 50 closely bound galaxies known as the Local Group, itself being part of the Virgo Supercluster. The Virgo Supercluster forms part of a greater structure, called Laniakea.[170]
Two smaller galaxies and a number of dwarf galaxies in the Local Group orbit the Milky Way. The largest of these is the Large Magellanic Cloud with a diameter of 14,000 light-years. It has a close companion, the Small Magellanic Cloud. The Magellanic Stream is a stream of neutral hydrogen gas extending from these two small galaxies across 100° of the sky. The stream is thought to have been dragged from the Magellanic Clouds in tidal interactions with the Milky Way.[171] Some of the dwarf galaxies orbiting the Milky Way are Canis Major Dwarf (the closest), Sagittarius Dwarf Elliptical Galaxy, Ursa Minor Dwarf, Sculptor Dwarf, Sextans Dwarf, Fornax Dwarf, and Leo I Dwarf. The smallest dwarf galaxies of the Milky Way are only 500 light-years in diameter. These include Carina Dwarf, Draco Dwarf, and Leo II Dwarf. There may still be undetected dwarf galaxies that are dynamically bound to the Milky Way, which is supported by the detection of nine new satellites of the Milky Way in a relatively small patch of the night sky in 2015.[172] There are also some dwarf galaxies that have already been absorbed by the Milky Way, such as Omega Centauri.[173]
In 2014 researchers reported that most satellite galaxies of the Milky Way actually lie in a huge disk, with almost all satellite galaxies moving in the same direction within this disk.[174] This came as a surprise: according to standard cosmology, the galaxies should form in halos of dark matter, be widely distributed and should move in random directions. This discrepancy is still not fully explained.[175]
In January 2006, researchers reported that the heretofore unexplained warp in the disk of the Milky Way has now been mapped and found to be a ripple or vibration set up by the Large and Small Magellanic Clouds as they orbit the Milky Way, causing vibrations when they pass through its edges. Previously, these two galaxies, at around 2% of the mass of the Milky Way, were considered too small to influence the Milky Way. However, in a computer model, the movement of these two galaxies creates a dark matter wake that amplifies their influence on the larger Milky Way.[176]
Current measurements suggest the Andromeda Galaxy is approaching us at 100 to 140 km/s (220,000 to 310,000 mph). In 3 to 4 billion years, there may be an Andromeda–Milky Way collision, depending on the importance of unknown lateral components to the galaxies' relative motion. If they collide, the chance of individual stars colliding with each other is extremely low, but instead the two galaxies will merge to form a single elliptical galaxy or perhaps a large disk galaxy[177] over the course of about a billion years.[178]
Velocity
Although special relativity states that there is no "preferred" inertial frame of reference in space with which to compare the Milky Way, the Milky Way does have a velocity with respect to cosmological frames of reference.
One such frame of reference is the Hubble flow, the apparent motions of galaxy clusters due to the expansion of space. Individual galaxies, including the Milky Way, have peculiar velocities relative to the average flow. Thus, to compare the Milky Way to the Hubble flow, one must consider a volume large enough so that the expansion of the Universe dominates over local, random motions. A large enough volume means that the mean motion of galaxies within this volume is equal to the Hubble flow. Astronomers believe the Milky Way is moving at approximately 630 km/s (1,400,000 mph) with respect to this local co-moving frame of reference.[179] The Milky Way is moving in the general direction of the Great Attractor and other galaxy clusters, including the Shapley supercluster, behind it.[180] The Local Group (a cluster of gravitationally bound galaxies containing, among others, the Milky Way and the Andromeda Galaxy) is part of a supercluster called the Local Supercluster, centered near the Virgo Cluster: although they are moving away from each other at 967 km/s (2,160,000 mph) as part of the Hubble flow, this velocity is less than would be expected given the 16.8 million pc distance due to the gravitational attraction between the Local Group and the Virgo Cluster.[181]
Another reference frame is provided by the cosmic microwave background (CMB). The Milky Way is moving at 552 ± 6 km/s (1,235,000 ± 13,000 mph)[17] with respect to the photons of the CMB, toward 10.5 right ascension, −24° declination (J2000 epoch, near the center of Hydra). This motion is observed by satellites such as the Cosmic Background Explorer (COBE) and the Wilkinson Microwave Anisotropy Probe (WMAP) as a dipole contribution to the CMB, as photons in equilibrium in the CMB frame get blue-shifted in the direction of the motion and red-shifted in the opposite direction.[17]
Etymology and mythology
In Babylonia, the Milky Way was said to be the tail of Tiamat, set in the sky by Marduk after he had slain the salt water goddess. It is believed this account, from the Enuma Elish had Marduk replace an earlier Sumerian story when Enlil of Nippur had slain the goddess.
In western culture the name "Milky Way" is derived from its appearance as a dim un-resolved "milky" glowing band arching across the night sky. The term is a translation of the Classical Latin via lactea, in turn derived from the Hellenistic Greek γαλαξίας, short for γαλαξίας κύκλος (galaxías kýklos, "milky circle"). The Ancient Greek γαλαξίας (galaxias) – from root γαλακτ-, γάλα ("milk") + -ίας (forming adjectives) – is also the root of "galaxy", the name for our, and later all such, collections of stars.[21][182][183][184] In Greek mythology it was supposedly made from the forceful suckling of Heracles, when Hera acted as a wetnurse for the hero.
The Milky Way, or "milk circle", was just one of 11 "circles" the Greeks identified in the sky, others being the zodiac, the meridian, the horizon, the equator, the tropics of Cancer and Capricorn, Arctic and Antarctic circles, and two colure circles passing through both poles.[185]
Astronomical history
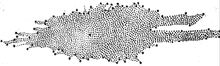
In Meteorologica (DK 59 A80), Aristotle (384–322 BC) wrote that the Greek philosophers Anaxagoras (c. 500–428 BC) and Democritus (460–370 BC) proposed that the Milky Way might consist of distant stars.[186] However, Aristotle himself believed the Milky Way to be caused by "the ignition of the fiery exhalation of some stars which were large, numerous and close together"[187] and that the "ignition takes place in the upper part of the atmosphere, in the region of the world which is continuous with the heavenly motions."[188][189] The Neoplatonist philosopher Olympiodorus the Younger (c. 495–570 A.D.) criticized this view, arguing that if the Milky Way were sublunary, it should appear different at different times and places on Earth, and that it should have parallax, which it does not. In his view, the Milky Way is celestial. This idea would be influential later in the Islamic world.[190]
The Persian astronomer Abū Rayhān al-Bīrūnī (973–1048) proposed that the Milky Way is "a collection of countless fragments of the nature of nebulous stars".[191] The Andalusian astronomer Avempace (d 1138) proposed the Milky Way to be made up of many stars but appears to be a continuous image due to the effect of refraction in Earth's atmosphere, citing his observation of a conjunction of Jupiter and Mars in 1106 or 1107 as evidence.[189] Ibn Qayyim Al-Jawziyya (1292–1350) proposed that the Milky Way is "a myriad of tiny stars packed together in the sphere of the fixed stars" and that these stars are larger than planets.[192]
According to Jamil Ragep, the Persian astronomer Naṣīr al-Dīn al-Ṭūsī (1201–1274) in his Tadhkira writes: "The Milky Way, i.e. the Galaxy, is made up of a very large number of small, tightly clustered stars, which, on account of their concentration and smallness, seem to be cloudy patches. Because of this, it was likened to milk in color."[193]
Actual proof of the Milky Way consisting of many stars came in 1610 when Galileo Galilei used a telescope to study the Milky Way and discovered that it is composed of a huge number of faint stars.[194][195] In a treatise in 1755, Immanuel Kant, drawing on earlier work by Thomas Wright,[196] speculated (correctly) that the Milky Way might be a rotating body of a huge number of stars, held together by gravitational forces akin to the Solar System but on much larger scales.[197] The resulting disk of stars would be seen as a band on the sky from our perspective inside the disk. Kant also conjectured that some of the nebulae visible in the night sky might be separate "galaxies" themselves, similar to our own. Kant referred to both the Milky Way and the "extragalactic nebulae" as "island universes", a term still current up to the 1930s.[198][199][200]
The first attempt to describe the shape of the Milky Way and the position of the Sun within it was carried out by William Herschel in 1785 by carefully counting the number of stars in different regions of the visible sky. He produced a diagram of the shape of the Milky Way with the Solar System close to the center.[201]
In 1845, Lord Rosse constructed a new telescope and was able to distinguish between elliptical and spiral-shaped nebulae. He also managed to make out individual point sources in some of these nebulae, lending credence to Kant's earlier conjecture.[202]

In 1917, Heber Curtis had observed the nova S Andromedae within the Great Andromeda Nebula (Messier object 31). Searching the photographic record, he found 11 more novae. Curtis noticed that these novae were, on average, 10 magnitudes fainter than those that occurred within the Milky Way. As a result, he was able to come up with a distance estimate of 150,000 parsecs. He became a proponent of the "island universes" hypothesis, which held that the spiral nebulae were actually independent galaxies.[203] In 1920 the Great Debate took place between Harlow Shapley and Heber Curtis, concerning the nature of the Milky Way, spiral nebulae, and the dimensions of the Universe. To support his claim that the Great Andromeda Nebula is an external galaxy, Curtis noted the appearance of dark lanes resembling the dust clouds in the Milky Way, as well as the significant Doppler shift.[204]
The controversy was conclusively settled by Edwin Hubble in the early 1920s using the Mount Wilson observatory 2.5 m (100 in) Hooker telescope. With the light-gathering power of this new telescope he was able to produce astronomical photographs that resolved the outer parts of some spiral nebulae as collections of individual stars. He was also able to identify some Cepheid variables that he could use as a benchmark to estimate the distance to the nebulae. He found that the Andromeda Nebula is 275,000 parsecs from the Sun, far too distant to be part of the Milky Way.[25][205]
Mapping
The satellite Gaia is mapping the Milky Way with five planned releases of the maps to the year 2022.[206][207]
See also
Notes
- ↑ Jay M. Pasachoff in his textbook Astronomy: From the Earth to the Universe states the term Milky Way should refer exclusively to the band of light that the galaxy forms in the night sky, while the galaxy should receive the full name Milky Way Galaxy. See:
- Pasachoff, Jay M. (1994). Astronomy: From the Earth to the Universe. Harcourt School. p. 500. ISBN 0-03-001667-3.
- ↑ See also Bortle Dark-Sky Scale.
- ↑ For a photo see: "Sagittarius A*: Milky Way monster stars in cosmic reality show". Chandra X-ray Observatory. Harvard-Smithsonian Center for Astrophysics. January 6, 2003. Retrieved May 20, 2012.
- ↑ Karachentsev et al. give a blue absolute magnitude of −20.8. Combined with a color index of 0.55 estimated here, an absolute visual magnitude of −21.35 (−20.8 − 0.55 = −21.35) is obtained. Note that determining the absolute magnitude of the Milky Way is very difficult, because Earth is inside it.
References
- 1 2 Gerhard, O. (2002). "Mass distribution in our Galaxy". Space Science Reviews. 100 (1/4): 129–138. arXiv:astro-ph/0203110
. Bibcode:2002astro.ph..3110G. doi:10.1023/A:1015818111633.
- ↑ Frommert, Hartmut; Kronberg, Christine (August 26, 2005). "Classification of the Milky Way Galaxy". SEDS. Retrieved 2015-05-30.
- ↑ Hall, Shannon (2015-05-04). "Size of the Milky Way Upgraded, Solving Galaxy Puzzle". Space.com. Retrieved 2015-06-09.
- 1 2 Coffey, Jeffrey. "How big is the Milky Way?". Universe Today. Archived from the original on September 24, 2013. Retrieved November 28, 2007.
- 1 2 Rix, Hans-Walter; Bovy, Jo (2013). "The Milky Way's Stellar Disk". The Astronomy and Astrophysics Review. in press. arXiv:1301.3168
. Bibcode:2013A&ARv..21...61R. doi:10.1007/s00159-013-0061-8.
- ↑ "NASA – Galaxy". NASA and World Book. Nasa.gov. November 29, 2007. Archived from the original on April 12, 2009. Retrieved December 6, 2012.
- ↑ Staff (December 16, 2008). "How Many Stars are in the Milky Way?". Universe Today. Retrieved August 10, 2010.
- ↑ Odenwald, S. (March 17, 2014). "Counting the Stars in the Milky Way". The Huffington Post. Retrieved June 9, 2014.
- 1 2 3 H.E. Bond; E. P. Nelan; D. A. VandenBerg; G. H. Schaefer; et al. (February 13, 2013). "HD 140283: A Star in the Solar Neighborhood that Formed Shortly After the Big Bang". The Astrophysical Journal. 765 (1): L12. arXiv:1302.3180
. Bibcode:2013ApJ...765L..12B. doi:10.1088/2041-8205/765/1/L12.
- 1 2 3 McMillan, P. J. (July 2011). "Mass models of the Milky Way". Monthly Notices of the Royal Astronomical Society. 414 (3): 2446–2457. arXiv:1102.4340
. Bibcode:2011MNRAS.414.2446M. doi:10.1111/j.1365-2966.2011.18564.x.
- ↑ Kafle, P.R.; Sharma, S.; Lewis, G.F.; Bland-Hawthorn, J. (2012). "Kinematics of the Stellar Halo and the Mass Distribution of the Milky Way Using Blue Horizontal Branch Stars". The Astrophysical Journal. 761 (2): 17. arXiv:1210.7527
. Bibcode:2012ApJ...761...98K. doi:10.1088/0004-637X/761/2/98.
- 1 2 3 Kafle, P.R.; Sharma, S.; Lewis, G.F.; Bland-Hawthorn, J. (2014). "On the Shoulders of Giants: Properties of the Stellar Halo and the Milky Way Mass Distribution". The Astrophysical Journal. 794 (1): 17. arXiv:1408.1787
. Bibcode:2014ApJ...794...59K. doi:10.1088/0004-637X/794/1/59.
- ↑ Karachentsev, Igor. "Double Galaxies §7.1". ned.ipac.caltech.edu. Izdatel'stvo Nauka. Retrieved 5 April 2015.
- 1 2 3 4 Gillessen, S.; et al. (2009). "Monitoring stellar orbits around the massive black hole in the Galactic Center". Astrophysical Journal. 692 (2): 1075–1109. arXiv:0810.4674
. Bibcode:2009ApJ...692.1075G. doi:10.1088/0004-637X/692/2/1075.
- 1 2 3 4 Sparke, Linda S.; Gallagher, John S. (2007). Galaxies in the Universe: An Introduction. p. 90. ISBN 9781139462389.
- 1 2 Gerhard, O. "Pattern speeds in the Milky Way". arXiv:1003.2489v1
.
- 1 2 3 Kogut, A.; et al. (1993). "Dipole anisotropy in the COBE differential microwave radiometers first-year sky maps". The Astrophysical Journal. 419: 1. arXiv:astro-ph/9312056
. Bibcode:1993ApJ...419....1K. doi:10.1086/173453.
- ↑ "Oxford Dictionaries: Milky Way". Oxford University Press. Archived from the original on May 8, 2013. Retrieved October 31, 2012.
- ↑ Merriam-Webster Incorporated. "Milky Way Galaxy". Merriam-Webster Incorporated. Retrieved October 31, 2012.
- ↑ Encyclopædia Britannica, Inc. "Milky Way Galaxy". Encyclopædia Britannica, Inc. Retrieved October 31, 2012.
- 1 2 Harper, Douglas. "galaxy". Online Etymology Dictionary. Retrieved May 20, 2012.
- ↑ Jankowski, Connie (2010). Pioneers of Light and Sound. Compass Point Books. p. 6. ISBN 0-7565-4306-1.
- ↑ Schiller, Jon (2010). Big Bang & Black Holes. CreateSpace. p. 163. ISBN 1-4528-6552-3.
- ↑ Shapley, H.; Curtis, H. D. (1921). "The Scale of the Universe". Bulletin of the National Research Council. 2: 171–217. Bibcode:1921BuNRC...2..171S.
- 1 2 Sandage, Allan (1989). "Edwin Hubble, 1889–1953". Journal of the Royal Astronomical Society of Canada. 83 (6). Bibcode:1989JRASC..83..351S.
- ↑ Gott III, J. R.; et al. (2005). "A Map of the Universe". Astrophysical Journal. 624 (2): 463–484. arXiv:astro-ph/0310571
. Bibcode:2005ApJ...624..463G. doi:10.1086/428890.
- ↑ Karl Hille, ed. (October 13, 2016). "Hubble Reveals Observable Universe Contains 10 Times More Galaxies Than Previously Thought". NASA. Retrieved October 17, 2016.
- ↑ Elizabeth Howell (January 20, 2015). "How Big Is The Milky Way?". Universe Today. Archived from the original on October 15, 2014.
- 1 2 Mary L. Martialay (March 11, 2015). "The Corrugated Galaxy—Milky Way May Be Much Larger Than Previously Estimated" (Press release). Rensselaer Polytechnic Institute. Archived from the original on March 13, 2015.
- ↑ "Milky Way". BBC. Archived from the original on March 2, 2012.
- ↑ "How Many Stars in the Milky Way?". NASA Blueshift.
- 1 2 3 Cassan, A.; et al. (January 11, 2012). "One or more bound planets per Milky Way star from microlensing observations". Nature. 481 (7380): 167–169. arXiv:1202.0903
. Bibcode:2012Natur.481..167C. doi:10.1038/nature10684. PMID 22237108.
- 1 2 Staff (January 2, 2013). "100 Billion Alien Planets Fill Our Milky Way Galaxy: Study". Space.com. Archived from the original on October 8, 2014. Retrieved January 3, 2013.
- 1 2 3 Koupelis, Theo; Kuhn, Karl F. (2007). In Quest of the Universe. Jones & Bartlett Publishers. p. 492; Figure 16-13. ISBN 0-7637-4387-9.
- ↑ "Laniakea: Our home supercluster". youtube.com.
- ↑ Tully, R. Brent; et al. (September 4, 2014). "The Laniakea supercluster of galaxies". Nature. 513 (7516): 71–73. arXiv:1409.0880
. Bibcode:2014Natur.513...71T. doi:10.1038/nature13674.
- ↑ Pasachoff, Jay M. (1994). Astronomy: From the Earth to the Universe. Harcourt School. p. 500. ISBN 0-03-001667-3.
- ↑ Rey, H. A. (1976). The Stars. Houghton Mifflin Harcourt. p. 145. ISBN 0395248302.
- ↑ Crumey, Andrew (2014). "Human contrast threshold and astronomical visibility". Monthly Notices of the Royal Astronomical Society. 442: 2600–2619. arXiv:1405.4209
. Bibcode:2014MNRAS.442.2600C. doi:10.1093/mnras/stu992.
- ↑ Steinicke, Wolfgang; Jakiel, Richard (2007). Galaxies and how to observe them. Astronomers' observing guides. Springer. p. 94. ISBN 1-85233-752-4.
- ↑ Falchi, Fabio; Cinzano, Pierantonio; Duriscoe, Dan; Kyba, Christopher C. M.; Elvidge, Christopher D.; Baugh, Kimberly; Portnov, Boris A.; Rybnikova, Nataliya A.; Furgoni, Riccardo (2016-06-01). "The new world atlas of artificial night sky brightness". Science Advances. 2 (6): e1600377. doi:10.1126/sciadv.1600377. ISSN 2375-2548. PMC 4928945
. PMID 27386582.
- ↑ The center of the Galaxy lies in the direction of the constellation Sagittarius. From Sagittarius, the hazy band of white light appears to pass westward through the constellations of Scorpius, Ara, Norma, Triangulum Australe, Circinus, Centaurus, Musca, Crux, Carina, Vela, Puppis, Canis Major, Monoceros, Orion and Gemini, Taurus, to the Galactic anticenter in Auriga. From there, it passes through Perseus, Andromeda, Cassiopeia, Cepheus and Lacerta, Cygnus, Vulpecula, Sagitta, Aquila, Ophiuchus, Scutum, and back to Sagittarius.
- ↑ de Vaucouleurs, G.; Pence, W. D. (October 1978). "An outsider's view of the Galaxy – Photometric parameters, scale lengths, and absolute magnitudes of the spheroidal and disk components of our Galaxy". Astronomical Journal. 83: 1163–1173. Bibcode:1978AJ.....83.1163D. doi:10.1086/112305.
- ↑ "How Big is Our Universe: How far is it across the Milky Way?". NASA-Smithsonian Education Forum on the Structure and Evolution of the Universe, at the Harvard Smithsonian Center for Astrophysics. Retrieved March 13, 2013.
- ↑ Newberg, Heidi Jo; et al. (March 1, 2015). "Rings and Radial Waves in the Disk of the Milky Way". The Astrophysical Journal. 801 (2): 105. arXiv:1503.00257
. Bibcode:2015ApJ...801..105X. doi:10.1088/0004-637X/801/2/105.
- ↑ Karachentsev, I. D.; Kashibadze, O. G. (2006). "Masses of the local group and of the M81 group estimated from distortions in the local velocity field". Astrophysics. 49 (1): 3–18. Bibcode:2006Ap.....49....3K. doi:10.1007/s10511-006-0002-6.
- ↑ Vayntrub, Alina (2000). "Mass of the Milky Way". The Physics Factbook. Archived from the original on August 13, 2014. Retrieved May 9, 2007.
- ↑ Battaglia, G.; et al. (2005). "The radial velocity dispersion profile of the Galactic halo: Constraining the density profile of the dark halo of the Milky Way". Monthly Notices of the Royal Astronomical Society. 364: 433–442. arXiv:astro-ph/0506102
. Bibcode:2005MNRAS.364..433B. doi:10.1111/j.1365-2966.2005.09367.x.
- ↑ Finley, Dave; Aguilar, David (January 5, 2009). "Milky Way a Swifter Spinner, More Massive, New Measurements Show" (Press release). National Radio Astronomy Observatory. Archived from the original on August 8, 2014. Retrieved January 20, 2009.
- ↑ Reid, M. J.; et al. (2009). "Trigonometric parallaxes of massive star-forming regions. VI. Galactic structure, fundamental parameters, and noncircular motions". The Astrophysical Journal. 700: 137–148. arXiv:0902.3913
. Bibcode:2009ApJ...700..137R. doi:10.1088/0004-637X/700/1/137.
- ↑ Gnedin, O. Y.; et al. (2010). "The mass profile of the Galaxy to 80 kpc". The Astrophysical Journal. 720: L108. arXiv:1005.2619
. Bibcode:2010ApJ...720L.108G. doi:10.1088/2041-8205/720/1/L108.
- 1 2 Peñarrubia, Jorge; et al. "A dynamical model of the local cosmic expansion". Monthly Notices of the Royal Astronomical Society. 433 (3): 2204–2022. arXiv:1405.0306
. Bibcode:2014MNRAS.443.2204P. doi:10.1093/mnras/stu879.
- ↑ Phelps, Steven; et al. (October 2013). "The Mass of the Milky Way and M31 Using the Method of Least Action". The Astrophysical Journal. 775 (2): 12. arXiv:1306.4013
. Bibcode:2013ApJ...775..102P. doi:10.1088/0004-637X/775/2/102. 102.
- ↑ Kafle, Prajwal Raj; et al. (October 2014). "On the Shoulders of Giants: Properties of the Stellar Halo and the Milky Way Mass Distribution". The Astrophysical Journal. 794 (1): 17. arXiv:1408.1787
. Bibcode:2014ApJ...794...59K. doi:10.1088/0004-637X/794/1/59. 59.
- ↑ Licquia, Timothy; Newman, J. (2013). "Improved Constraints on the Total Stellar Mass, Color, and Luminosity of the Milky Way". American Astronomical Society, AAS Meeting #221, #254.11. Bibcode:2013AAS...22125411L.
- 1 2 3 "The Interstellar Medium". Retrieved 2 May 2015.
- 1 2 "Lecture Seven: The Milky Way: Gas" (PDF). Retrieved 2 May 2015.
- ↑ Frommert, H.; Kronberg, C. (August 25, 2005). "The Milky Way Galaxy". SEDS. Retrieved May 9, 2007.
- ↑ Wethington, Nicholos. "How Many Stars are in the Milky Way?". Retrieved April 9, 2010.
- ↑ Villard, Ray (January 11, 2012). "The Milky Way Contains at Least 100 Billion Planets According to Survey". HubbleSite.org. Archived from the original on July 23, 2014. Retrieved January 11, 2012.
- ↑ Young, Kelly (June 6, 2006). "Andromeda Galaxy hosts a trillion stars". NewScientist. Retrieved June 8, 2006.
- 1 2 Levine, E. S.; Blitz, L.; Heiles, C. (2006). "The spiral structure of the outer Milky Way in hydrogen". Science. 312 (5781): 1773–1777. arXiv:astro-ph/0605728
. Bibcode:2006Sci...312.1773L. doi:10.1126/science.1128455. PMID 16741076.
- ↑ Dickey, J. M.; Lockman, F. J. (1990). "H I in the Galaxy". Annual Review of Astronomy and Astrophysics. 28: 215–259. Bibcode:1990ARA&A..28..215D. doi:10.1146/annurev.aa.28.090190.001243.
- ↑ Savage, B. D.; Wakker, B. P. (2009). "The extension of the transition temperature plasma into the lower galactic halo". The Astrophysical Journal. 702 (2): 1472–1489. arXiv:0907.4955
. Bibcode:2009ApJ...702.1472S. doi:10.1088/0004-637X/702/2/1472.
- ↑ Sale, S. E.; et al. (2010). "The structure of the outer Galactic disc as revealed by IPHAS early A stars". Monthly Notices of the Royal Astronomical Society. 402 (2): 713–723. arXiv:0909.3857
. Bibcode:2010MNRAS.402..713S. doi:10.1111/j.1365-2966.2009.15746.x.
- ↑ Connors, Tim W.; Kawata, Daisuke; Gibson, Brad K. (2006). "N-body simulations of the Magellanic stream". Monthly Notices of the Royal Astronomical Society. 371 (1): 108–120. arXiv:astro-ph/0508390
. Bibcode:2006MNRAS.371..108C. doi:10.1111/j.1365-2966.2006.10659.x.
- ↑ Coffey, Jerry. "Absolute Magnitude". Archived from the original on September 13, 2011.
- ↑ Karachentsev, Igor D.; Karachentseva, Valentina E.; Huchtmeier, Walter K.; Makarov, Dmitry I. (2003). "A Catalog of Neighboring Galaxies". The Astronomical Journal. 127 (4): 2031–2068. Bibcode:2004AJ....127.2031K. doi:10.1086/382905.
- ↑ Borenstein, Seth (February 19, 2011). "Cosmic census finds crowd of planets in our galaxy". The Washington Post. Associated Press. Archived from the original on February 21, 2011.
- ↑ Sumi, T.; et al. (2011). "Unbound or distant planetary mass population detected by gravitational microlensing". Nature. 473 (7347): 349–352. arXiv:1105.3544
. Bibcode:2011Natur.473..349S. doi:10.1038/nature10092. PMID 21593867.
- ↑ "Free-Floating Planets May be More Common Than Stars". Pasadena, CA: NASA's Jet Propulsion Laboratory. February 18, 2011. Archived from the original on May 25, 2011.
The team estimates there are about twice as many of them as stars.
- ↑ Staff (January 7, 2013). "17 Billion Earth-Size Alien Planets Inhabit Milky Way". Space.com. Archived from the original on October 6, 2014. Retrieved January 8, 2013.
- 1 2 Overbye, Dennis (November 4, 2013). "Far-Off Planets Like the Earth Dot the Galaxy". New York Times. Retrieved November 5, 2013.
- 1 2 Petigura, Eric A.; Howard, Andrew W.; Marcy, Geoffrey W. (October 31, 2013). "Prevalence of Earth-size planets orbiting Sun-like stars". Proceedings of the National Academy of Sciences of the United States of America. 110: 19273–19278. arXiv:1311.6806
. Bibcode:2013PNAS..11019273P. doi:10.1073/pnas.1319909110. Retrieved November 5, 2013.
- ↑ Borenstein, Seth (November 4, 2013). "Milky Way Teeming With Billions Of Earth-Size Planets". The Associated Press. The Huffington Post.
- ↑ Khan, Amina (November 4, 2013). "Milky Way may host billions of Earth-size planets". Los Angeles Times. Retrieved November 5, 2013.
- ↑ Staff (January 7, 2013). "'Exocomets' Common Across Milky Way Galaxy". Space.com. Archived from the original on September 16, 2014. Retrieved January 8, 2013.
- 1 2 3 Benjamin, R. A. (2008). Beuther, H.; Linz, H.; Henning, T., eds. The Spiral Structure of the Galaxy: Something Old, Something New... Massive Star Formation: Observations Confront Theory. 387. Astronomical Society of the Pacific Conference Series. p. 375. Bibcode:2008ASPC..387..375B.
See also Bryner, Jeanna (June 3, 2008). "New Images: Milky Way Loses Two Arms". Space.com. Retrieved June 4, 2008. - 1 2 Chou, Felicia; Anderson, Janet; Watzke, Megan (January 5, 2015). "RELEASE 15-001 – NASA's Chandra Detects Record-Breaking Outburst from Milky Way's Black Hole". NASA. Retrieved January 6, 2015.
- ↑ Chen, W.; Gehrels, N.; Diehl, R.; Hartmann, D. (1996). "On the spiral arm interpretation of COMPTEL ^26^Al map features". Space Science Reviews. 120: 315–316. Bibcode:1996A&AS..120C.315C.
- ↑ McKee, Maggie (August 16, 2005). "Bar at Milky Way's heart revealed". New Scientist. Archived from the original on October 9, 2014. Retrieved June 17, 2009.
- ↑ Blaauw, A.; et al. (1960), "The new I. A. U. system of galactic coordinates (1958 revision)", Monthly Notices of the Royal Astronomical Society, 121 (2): 123–131, Bibcode:1960MNRAS.121..123B, doi:10.1093/mnras/121.2.123
- 1 2 Wilson, Thomas L.; et al. (2009), Tools of Radio Astronomy, Springer Science & Business Media, ISBN 3540851216
- 1 2 Kiss, Cs; Moór, A.; Tóth, L. V. (April 2004). "Far-infrared loops in the 2nd Galactic Quadrant". Astronomy and Astrophysics. 418: 131–141. arXiv:astro-ph/0401303
. Bibcode:2004A&A...418..131K. doi:10.1051/0004-6361:20034530.
- 1 2 Lampton, M., Lieu, R.; et al. (February 1997). "An All-Sky Catalog of Faint Extreme Ultraviolet Sources". The Astrophysical Journal Supplement Series. 108 (2): 545–557. Bibcode:1997ApJS..108..545L. doi:10.1086/312965.
- ↑ van Woerden, Hugo; Strom, Richard G. (June 2006). "The beginnings of radio astronomy in the Netherlands" (PDF). Journal of Astronomical History and Heritage. 9 (1): 3–20. Bibcode:2006JAHH....9....3V.
- 1 2 3 4 Ghez, A. M.; et al. (December 2008). "Measuring distance and properties of the Milky Way's central supermassive black hole with stellar orbits". The Astrophysical Journal. 689 (2): 1044–1062. arXiv:0808.2870
. Bibcode:2008ApJ...689.1044G. doi:10.1086/592738.
- ↑ Reid, M. J.; et al. (November 2009). "A trigonometric parallax of Sgr B2". The Astrophysical Journal. 705 (2): 1548–1553. arXiv:0908.3637
. Bibcode:2009ApJ...705.1548R. doi:10.1088/0004-637X/705/2/1548.
- 1 2 Vanhollebeke, E.; Groenewegen, M. A. T.; Girardi, L. (April 2009). "Stellar populations in the Galactic bulge. Modelling the Galactic bulge with TRILEGAL". Astronomy and Astrophysics. 498: 95–107. Bibcode:2009A&A...498...95V. doi:10.1051/0004-6361/20078472.
- 1 2 3 4 Majaess, D. (March 2010). "Concerning the Distance to the Center of the Milky Way and Its Structure". Acta Astronomica. 60 (1): 55. arXiv:1002.2743
. Bibcode:2010AcA....60...55M.
- ↑ Grant, J.; Lin, B. (2000). "The Stars of the Milky Way". Fairfax Public Access Corporation. Retrieved May 9, 2007.
- ↑ Shen, J.; Rich, R. M.; Kormendy, J.; Howard, C. D.; De Propris, R.; Kunder, A. (2010). "Our Milky Way As a Pure-Disk Galaxy—A Challenge for Galaxy Formation". The Astrophysical Journal. 720: L72. arXiv:1005.0385
. Bibcode:2010ApJ...720L..72S. doi:10.1088/2041-8205/720/1/L72.
- ↑ Jones, Mark H.; Lambourne, Robert J.; Adams, David John (2004). An Introduction to Galaxies and Cosmology. Cambridge University Press. pp. 50–51. ISBN 0-521-54623-0.
- 1 2 Wang, Q. D.; Nowak, M. A.; Markoff, S. B.; Baganoff, F. K.; Nayakshin, S.; Yuan, F.; Cuadra, J.; Davis, J.; Dexter, J.; Fabian, A. C.; Grosso, N.; Haggard, D.; Houck, J.; Ji, L.; Li, Z.; Neilsen, J.; Porquet, D.; Ripple, F.; Shcherbakov, R. V. (2013). "Dissecting X-ray-Emitting Gas Around the Center of Our Galaxy". Science. 341 (6149): 981–983. arXiv:1307.5845
. Bibcode:2013Sci...341..981W. doi:10.1126/science.1240755. PMID 23990554.
- ↑ Blandford, R. D. (8–12 August 1998). Origin and Evolution of Massive Black Holes in Galactic Nuclei. Galaxy Dynamics, proceedings of a conference held at Rutgers University, ASP Conference Series. 182. Rutgers University (published August 1999). arXiv:astro-ph/9906025
. Bibcode:1999ASPC..182...87B.
- ↑ Frolov, Valeri P.; Zelnikov, Andrei (2011). Introduction to Black Hole Physics. Oxford University Press. pp. 11, 36. ISBN 0199692297.
- ↑ Cabrera-Lavers, A.; et al. (December 2008). "The long Galactic bar as seen by UKIDSS Galactic plane survey". Astronomy and Astrophysics. 491 (3): 781–787. arXiv:0809.3174
. Bibcode:2008A&A...491..781C. doi:10.1051/0004-6361:200810720.
- ↑ Nishiyama, S.; et al. (2005). "A distinct structure inside the Galactic bar". The Astrophysical Journal. 621 (2): L105. arXiv:astro-ph/0502058
. Bibcode:2005ApJ...621L.105N. doi:10.1086/429291.
- ↑ Alcock, C.; et al. (1998). "The RR Lyrae population of the Galactic Bulge from the MACHO database: mean colors and magnitudes". The Astrophysical Journal. 492 (2): 190–199. arXiv:astro-ph/0502058
. Bibcode:2005ApJ...621L.105N. doi:10.1086/305017.
- ↑ Kunder, A.; Chaboyer, B. (2008). "Metallicity analysis of Macho Galactic Bulge RR0 Lyrae stars from their light curves". The Astronomical Journal. 136 (6): 2441–2452. arXiv:0809.1645
. Bibcode:2008AJ....136.2441K. doi:10.1088/0004-6256/136/6/2441.
- ↑ Staff (September 12, 2005). "Introduction: Galactic Ring Survey". Boston University. Retrieved May 10, 2007.
- ↑ Bhat, C. L.; Kifune, T.; Wolfendale, A. W. (November 21, 1985). "A cosmic-ray explanation of the galactic ridge of cosmic X-rays". Nature. 318 (6043): 267–269. Bibcode:1985Natur.318..267B. doi:10.1038/318267a0.
- ↑ Overbye, Dennis (November 9, 2010). "Bubbles of Energy Are Found in Galaxy". The New York Times.
- ↑ "NASA's Fermi Telescope Finds Giant Structure in our Galaxyl". NASA. Archived from the original on August 23, 2014. Retrieved November 10, 2010.
- ↑ Carretti, E.; Crocker, R. M.; Staveley-Smith, L.; Haverkorn, M.; Purcell, C.; Gaensler, B. M.; Bernardi, G.; Kesteven, M. J.; Poppi, S. (2013). "Giant magnetized outflows from the centre of the Milky Way". Nature. 493 (7430): 66–69. arXiv:1301.0512
. Bibcode:2013Natur.493...66C. doi:10.1038/nature11734. PMID 23282363.
- ↑ Churchwell, E.; et al. (2009). "The Spitzer/GLIMPSE surveys: a new view of the Milky Way". Publications of the Astronomical Society of the Pacific. 121 (877): 213–230. Bibcode:2009PASP..121..213C. doi:10.1086/597811.
- ↑ Taylor, J. H.; Cordes, J. M. (1993). "Pulsar distances and the galactic distribution of free electrons". The Astrophysical Journal. 411: 674. Bibcode:1993ApJ...411..674T. doi:10.1086/172870.
- 1 2 3 Russeil, D. (2003). "Star-forming complexes and the spiral structure of our Galaxy". Astronomy and Astrophysics. 397: 133–146. Bibcode:2003A&A...397..133R. doi:10.1051/0004-6361:20021504.
- ↑ Dame, T. M.; Hartmann, D.; Thaddeus, P. (2001). "The Milky Way in Molecular Clouds: A New Complete CO Survey". The Astrophysical Journal. 547 (2): 792–813. arXiv:astro-ph/0009217
. Bibcode:2001ApJ...547..792D. doi:10.1086/318388.
- 1 2 3 Majaess, D. J.; Turner, D. G.; Lane, D. J. (2009). "Searching Beyond the Obscuring Dust Between the Cygnus-Aquila Rifts for Cepheid Tracers of the Galaxy's Spiral Arms". The Journal of the American Association of Variable Star Observers. 37: 179. arXiv:0909.0897
. Bibcode:2009JAVSO..37..179M.
- ↑ Lépine, J. R. D.; et al. (2011). "The spiral structure of the Galaxy revealed by CS sources and evidence for the 4:1 resonance". Monthly Notices of the Royal Astronomical Society. 414 (2): 1607–1616. arXiv:1010.1790
. Bibcode:2011MNRAS.414.1607L. doi:10.1111/j.1365-2966.2011.18492.x.
- 1 2 Drimmel, R. (2000). "Evidence for a two-armed spiral in the Milky Way". Astronomy & Astrophysics. 358: L13–L16. arXiv:astro-ph/0005241
. Bibcode:2000A&A...358L..13D.
- 1 2 McClure-Griffiths, N. M.; Dickey, J. M.; Gaensler, B. M.; Green, A. J. (2004). "A Distant Extended Spiral Arm in the Fourth Quadrant of the Milky Way". The Astrophysical Journal. 607 (2): L127. arXiv:astro-ph/0404448
. Bibcode:2004ApJ...607L.127M. doi:10.1086/422031.
- ↑ Benjamin, R. A.; et al. (2005). "First GLIMPSE results on the stellar structure of the Galaxy". The Astrophysical Journal. 630 (2): L149–L152. arXiv:astro-ph/0508325
. Bibcode:2005ApJ...630L.149B. doi:10.1086/491785.
- ↑ "Massive stars mark out Milky Way's 'missing' arms", University of Leeds. December 17, 2013. Retrieved December 18, 2013.
- ↑ Westerholm, Russell (December 18, 2013). "Milky Way Galaxy Has Four Arms, Reaffirming Old Data and Contradicting Recent Research". University Herald. Retrieved December 18, 2013.
- 1 2 Urquhart, J. S.; Figura, C. C.; Moore, T. J. T.; Hoare, M. G.; et al. (January 2014). "The RMS Survey: Galactic distribution of massive star formation". Monthly Notices of the Royal Astronomical Society. 437 (2): 1791–1807. arXiv:1310.4758
. Bibcode:2014MNRAS.437.1791U. doi:10.1093/mnras/stt2006.
- ↑ Expansion d'une structure spirale dans le noyau du Système Galactique, et position de la radiosource Sagittarius A, Comptes Rendus l'Academie des Sciences, Vol. 244, p. 1691-1695, 1957
- 1 2 Dame, T. M.; Thaddeus, P. "A New Spiral Arm of the Galaxy: The Far 3-Kpc Arm". The Astrophysical Journal. 683 (2): L143−L146. arXiv:0807.1752
. Bibcode:2008ApJ...683L.143D. doi:10.1086/591669.
- ↑ "Milky Way's Inner Beauty Revealed". Harvard-Smithsonian Center for Astrophysics. June 3, 2008. Retrieved 2015-07-07.
- ↑ Matson, John (September 14, 2011). "Star-Crossed: Milky Way's Spiral Shape May Result from a Smaller Galaxy's Impact". Scientific American. Retrieved 2015-07-07.
- ↑ Mel'Nik, A.; Rautiainen, A. (2005). "Kinematics of the outer pseudorings and the spiral structure of the Galaxy". Astronomy Letters. 35 (9): 609–624. arXiv:0902.3353
. Bibcode:2009AstL...35..609M. doi:10.1134/s1063773709090047.
- ↑ Mel'Nik, A. (2005). "Outer pseudoring in the galaxy". Astronomische Nachrichten. 326: 599. arXiv:astro-ph/0510569
. Bibcode:2005AN....326Q.599M. doi:10.1002/asna.200585006.
- ↑ Lopez-Corredoira, M.; et al. (July 2012). "Comments on the "Monoceros" affair". arXiv:1207.2749
. Bibcode:2012arXiv1207.2749L.
- ↑ Harris, William E. (February 2003). "Catalog of Parameters for Milky Way Globular Clusters: The Database" (text). SEDS. Retrieved May 10, 2007.
- ↑ Dauphole, B.; et al. (September 1996). "The kinematics of globular clusters, apocentric distances and a halo metallicity gradient". Astronomy and Astrophysics. 313: 119–128. Bibcode:1996A&A...313..119D.
- ↑ Gnedin, O. Y.; Lee, H. M.; Ostriker, J. P. (1999). "Effects of Tidal Shocks on the Evolution of Globular Clusters". The Astrophysical Journal. 522 (2): 935–949. arXiv:astro-ph/9806245
. Bibcode:1999ApJ...522..935G. doi:10.1086/307659.
- ↑ Janes, K.A.; Phelps, R.L. (1980). "The galactic system of old star clusters: The development of the galactic disk". The Astronomical Journal. 108: 1773–1785. Bibcode:1994AJ....108.1773J. doi:10.1086/117192.
- ↑ Ibata, R.; et al. (2005). "On the accretion origin of a vast extended stellar disk around the Andromeda Galaxy". The Astrophysical Journal. 634 (1): 287–313. arXiv:astro-ph/0504164
. Bibcode:2005ApJ...634..287I. doi:10.1086/491727.
- ↑ "Outer Disk Ring?". SolStation. Retrieved May 10, 2007.
- ↑ T.M. Dame; P. Thaddeus (2011). "A Molecular Spiral Arm in the Far Outer Galaxy". The Astrophysical Journal. 734: L24. arXiv:1105.2523
. Bibcode:2011ApJ...734L..24D. doi:10.1088/2041-8205/734/1/l24.
- ↑ Jurić, M.; et al. (February 2008). "The Milky Way Tomography with SDSS. I. Stellar Number Density Distribution". The Astrophysical Journal. 673 (2): 864–914. arXiv:astro-ph/0510520
. Bibcode:2008ApJ...673..864J. doi:10.1086/523619.
- ↑ Boen, Brooke. "NASA's Chandra Shows Milky Way is Surrounded by Halo of Hot Gas09.24.12". Brooke Boen. Retrieved October 28, 2012.
- ↑ Gupta, A.; Mathur, S.; Krongold, Y.; Nicastro, F.; Galeazzi, M. (2012). "A Huge Reservoir of Ionized Gas Around the Milky Way: Accounting for the Missing Mass?". The Astrophysical Journal. 756: L8. arXiv:1205.5037
. Bibcode:2012ApJ...756L...8G. doi:10.1088/2041-8205/756/1/L8.
- ↑ "Galactic Halo: Milky Way is Surrounded by Huge Halo of Hot Gas". Smithsonian Astrophysical Observatory. September 24, 2012.
- ↑ Communications, Discovery. "OUR GALAXY SWIMS INSIDE A GIANT POOL OF HOT GAS". Discovery Communications. Retrieved October 28, 2012.
- 1 2 J.D. Harrington; Janet Anderson; Peter Edmonds (September 24, 2012). "NASA's Chandra Shows Milky Way is Surrounded by Halo of Hot Gas". NASA.
- ↑ Reid, M. J. (1993). "The distance to the center of the Galaxy". Annual Review of Astronomy and Astrophysics. 31: 345–372. Bibcode:1993ARA&A..31..345R. doi:10.1146/annurev.aa.31.090193.002021.
- ↑ Majaess, D. J.; Turner, D. G.; Lane, D. J. (2009). "Characteristics of the Galaxy according to Cepheids". Monthly Notices of the Royal Astronomical Society. 398 (1): 263–270. arXiv:0903.4206
. Bibcode:2009MNRAS.398..263M. doi:10.1111/j.1365-2966.2009.15096.x.
- ↑ English, Jayanne (January 14, 2000). "Exposing the Stuff Between the Stars". Hubble News Desk. Retrieved May 10, 2007.
- ↑ Peter Schneider (2006). Extragalactic Astronomy and Cosmology. Springer. p. 4, Figure 1.4. ISBN 3-540-33174-3.
- ↑ Jones, Mark H.; Lambourne, Robert J.; Adams, David John (2004). An Introduction to Galaxies and Cosmology. Cambridge University Press. p. 21; Figure 1.13. ISBN 0-521-54623-0.
- ↑ "Magnitude". National Solar Observatory—Sacramento Peak. Archived from the original on February 6, 2008. Retrieved August 9, 2013.
- ↑ Gillman, M.; Erenler, H. (2008). "The galactic cycle of extinction". International Journal of Astrobiology. 7. Bibcode:2008IJAsB...7...17G. doi:10.1017/S1473550408004047.
- ↑ Overholt, A. C.; Melott, A. L.; Pohl, M. (2009). "Testing the link between terrestrial climate change and galactic spiral arm transit". The Astrophysical Journal. 705 (2): L101–L103. arXiv:0906.2777
. Bibcode:2009ApJ...705L.101O. doi:10.1088/0004-637X/705/2/L101.
- ↑ Garlick, Mark Antony (2002). The Story of the Solar System. Cambridge University. p. 46. ISBN 0-521-80336-5.
- ↑ "Solar System's "Nose" Found; Aimed at Constellation Scorpius".
- ↑ Imamura, Jim (August 10, 2006). "Mass of the Milky Way Galaxy". University of Oregon. Archived from the original on March 1, 2007. Retrieved May 10, 2007.
- ↑ Peter Schneider (2006). Extragalactic Astronomy and Cosmology. Springer. p. 413. ISBN 3-540-33174-3.
- ↑ Wethington, Nicholas (May 27, 2009). "Formation of the Milky Way". Universe Today. Archived from the original on August 17, 2014.
- 1 2 Buser, R. (2000). "The Formation and Early Evolution of the Milky Way Galaxy". Science. 287 (5450): 69–74. Bibcode:2000Sci...287...69B. doi:10.1126/science.287.5450.69. PMID 10615051.
- ↑ Wakker, B. P.; Van Woerden, H. (1997). "High-Velocity Clouds". Annual Review of Astronomy and Astrophysics. 35: 217–266. Bibcode:1997ARA&A..35..217W. doi:10.1146/annurev.astro.35.1.217.
- ↑ Lockman, F. J.; et al. (2008). "The Smith Cloud: A High-Velocity Cloud Colliding with the Milky Way". The Astrophysical Journal. 679: L21–L24. arXiv:0804.4155
. Bibcode:2008ApJ...679L..21L. doi:10.1086/588838.
- ↑ Yin, J.; Hou, J.L; Prantzos, N.; Boissier, S.; et al. (2009). "Milky Way versus Andromeda: a tale of two disks". Astronomy and Astrophysics. 505 (2): 497–508. arXiv:0906.4821
. Bibcode:2009A&A...505..497Y. doi:10.1051/0004-6361/200912316.
- ↑ Hammer, F.; Puech, M.; Chemin, L.; Flores, H.; et al. (2007). "The Milky Way, an Exceptionally Quiet Galaxy: Implications for the Formation of Spiral Galaxies". The Astrophysical Journal. 662 (1): 322–334. arXiv:astro-ph/0702585
. Bibcode:2007ApJ...662..322H. doi:10.1086/516727.
- ↑ Mutch, S.J.; Croton, D.J.; Poole, G.B. (2011). "The Mid-life Crisis of the Milky Way and M31". The Astrophysical Journal. 736 (2): 84. arXiv:1105.2564
. Bibcode:2011ApJ...736...84M. doi:10.1088/0004-637X/736/2/84.
- ↑ Licquia, T.; Newman, J.A.; Poole, G.B. (2012). "What Is The Color Of The Milky Way?". American Astronomical Society. Bibcode:2012AAS...21925208L.
- ↑ "A firestorm of star birth (artist's illustration)". www.spacetelescope.org. ESA/Hubble. Retrieved 14 April 2015.
- ↑ Cayrel; et al. (2001). "Measurement of stellar age from uranium decay". Nature. 409: 691–692. arXiv:astro-ph/0104357
. Bibcode:2001Natur.409..691C. doi:10.1038/35055507.
- ↑ Cowan, J. J.; Sneden, C.; Burles, S.; Ivans, I. I.; Beers, T. C.; Truran, J. W.; Lawler, J. E.; Primas, F.; Fuller, G. M.; et al. (2002). "The Chemical Composition and Age of the Metal‐poor Halo Star BD +17o3248". The Astrophysical Journal. 572 (2): 861–879. arXiv:astro-ph/0202429
. Bibcode:2002ApJ...572..861C. doi:10.1086/340347.
- ↑ Krauss, L. M.; Chaboyer, B. (2003). "Age Estimates of Globular Clusters in the Milky Way: Constraints on Cosmology". Science. 299 (5603): 65–69. Bibcode:2003Sci...299...65K. doi:10.1126/science.1075631. PMID 12511641.
- 1 2 Frebel, A.; et al. (2007). "Discovery of HE 1523-0901, a strongly r-process-enhanced metal-poor star with detected uranium". The Astrophysical Journal. 660 (2): L117. arXiv:astro-ph/0703414
. Bibcode:2007ApJ...660L.117F. doi:10.1086/518122.
- ↑ "Hubble Finds Birth Certificate of Oldest Known Star in the Milky Way". NASA. March 7, 2013. Archived from the original on August 11, 2014.
- ↑ del Peloso, E. F. (2005). "The age of the Galactic thin disk from Th/Eu nucleocosmochronology. III. Extended sample". Astronomy and Astrophysics. 440 (3): 1153–1159. arXiv:astro-ph/0506458
. Bibcode:2005A&A...440.1153D. doi:10.1051/0004-6361:20053307.
- ↑ Skibba, Ramon (2016), "Milky Way retired early from star making" (New Scientist, 5 Mar, 2016), p.9
- ↑ Lynden-Bell, D. (1976-03-01). "Dwarf Galaxies and Globular Clusters in High Velocity Hydrogen Streams". Monthly Notices of the Royal Astronomical Society. 174 (3): 695–710. doi:10.1093/mnras/174.3.695. ISSN 0035-8711.
- ↑ Kroupa, P., C. Theis and C. M. Boily (2005) "The great disk of Milky-Way satellites and cosmological sub-structures" (Astronomy and Astrophysics, Volume 431, Number 2, February IV 2005) pp. 517 – 521
- ↑ Ibata, RA, GF Lewis, AR Conn, MJ Irwin (2013) "A vast, thin plane of corotating dwarf galaxies orbiting the Andromeda galaxy" (Nature 493, 62–65 (03 January 2013))
- ↑ Clarke, Stuart (2016), Smash and Grab: The Milky Way Dwarf satellites were violently acquired" (New Scientist No 3067, 2, April 2016) pp30-33
- ↑ R. Brent Tully; Helene Courtois; Yehuda Hoffman; Daniel Pomarède (September 2, 2014). "The Laniakea supercluster of galaxies". Nature (published September 4, 2014). 513 (7516): 71–73. arXiv:1409.0880
. Bibcode:2014Natur.513...71T. doi:10.1038/nature13674.
- ↑ Putman, M. E.; Staveley‐Smith, L.; Freeman, K. C.; Gibson, B. K.; Barnes, D. G. (2003). "The Magellanic Stream, High‐Velocity Clouds, and the Sculptor Group". The Astrophysical Journal. 586: 170–194. arXiv:astro-ph/0209127
. Bibcode:2003ApJ...586..170P. doi:10.1086/344477.
- ↑ Sergey E. Koposov; Vasily Belokurov; Gabriel Torrealba; N. Wyn Evans (10 March 2015). "Beasts of the Southern Wild. Discovery of a large number of Ultra Faint satellites in the vicinity of the Magellanic Clouds". The Astrophysical Journal. 805: 130. arXiv:1503.02079
. Bibcode:2015ApJ...805..130K. doi:10.1088/0004-637X/805/2/130.
- ↑ Noyola, E.; Gebhardt, K.; Bergmann, M. (April 2008). "Gemini and Hubble Space Telescope Evidence for an Intermediate-Mass Black Hole in ω Centauri". The Astrophysical Journal. 676 (2): 1008–1015. arXiv:0801.2782
. Bibcode:2008ApJ...676.1008N. doi:10.1086/529002.
- ↑ Lea Kivivali (11 June 2014). "Nearby satellite galaxies challenge standard model of galaxy formation". Swinburne University of Technology.
- ↑ Pawlowski; et al. (10 June 2014). "Co-orbiting satellite galaxy structures are still in conflict with the distribution of primordial dwarf galaxies". Monthly Notices of the Royal Astronomical Society. 442: 2362–2380. arXiv:1406.1799
. Bibcode:2014MNRAS.442.2362P. doi:10.1093/mnras/stu1005.
- ↑ "Milky Way Galaxy is warped and vibrating like a drum" (Press release). University of California, Berkeley. January 9, 2006. Archived from the original on July 16, 2014. Retrieved October 18, 2007.
- ↑ Junko Ueda; et al. "Cold molecular gas in merger remnants. I. Formation of molecular gas disks". The Astrophysical Journal Supplement Series. 214 (1). arXiv:1407.6873
. Bibcode:2014ApJS..214....1U. doi:10.1088/0067-0049/214/1/1.
- ↑ Wong, Janet (April 14, 2000). "Astrophysicist maps out our own galaxy's end". University of Toronto. Archived from the original on January 8, 2007. Retrieved January 11, 2007.
- ↑ Mark H. Jones; Robert J. Lambourne; David John Adams (2004). An Introduction to Galaxies and Cosmology. Cambridge University Press. p. 298. ISBN 0-521-54623-0.
- ↑ Kocevski, D. D.; Ebeling, H. (2006). "On the origin of the Local Group's peculiar velocity". The Astrophysical Journal. 645 (2): 1043–1053. arXiv:astro-ph/0510106
. Bibcode:2006ApJ...645.1043K. doi:10.1086/503666.
- ↑ Peirani, S; Defreitaspacheco, J (2006). "Mass determination of groups of galaxies: Effects of the cosmological constant". New Astronomy. 11 (4): 325–330. arXiv:astro-ph/0508614
. Bibcode:2006NewA...11..325P. doi:10.1016/j.newast.2005.08.008.
- ↑ Jankowski, Connie (2010). Pioneers of Light and Sound. Compass Point Books. p. 6. ISBN 0-7565-4306-1.
- ↑ Schiller, Jon (2010). Big Bang & Black Holes. CreateSpace. p. 163. ISBN 1-4528-6552-3.
- ↑ Simpson, John; Weiner, Edmund, eds. (March 30, 1989). The Oxford English Dictionary (2nd ed.). Oxford University Press. ISBN 0198611862. See the entries for "Milky Way" and "galaxy".
- ↑ Eratosthenes (1997). Condos, Theony, ed. Star Myths of the Greeks and Romans: A Sourcebook Containing the Constellations of Pseudo-Eratosthenes and the Poetic Astronomy of Hyginus. Red Wheel/Weiser. ISBN 1890482935.
- ↑ Aristotle with W. D. Ross, ed., The Works of Aristotle … (Oxford, England: Clarendon Press, 1931), vol. III, Meteorologica, E. W. Webster, trans., Book 1, Part 8, pp. 39–40 : "(2) Anaxagoras, Democritus, and their schools say that the milky way is the light of certain stars."
- ↑ (Aristotle with Ross, 1931), p. 41: "For it is natural to suppose that, if the motion of a single star excites a flame, that of all the stars should have a similar result, and especially in that region in which the stars are biggest and most numerous and nearest to one another."
- ↑ (Aristotle with Ross, 1931), p. 43 : "We have now explained the phenomena that occur in that part of the terrestrial world which is continuous with the motions of the heavens, namely, shooting-stars and the burning flame, comets and the milky way, these being the chief affections that appear in that region."
- 1 2 Montada, Josep Puig (September 28, 2007). "Ibn Bajja". Stanford Encyclopedia of Philosophy. Retrieved July 11, 2008.
- ↑ Heidarzadeh, Tofigh (2008). A history of physical theories of comets, from Aristotle to Whipple. Springer. pp. 23–25. ISBN 1-4020-8322-X.
- ↑ O'Connor, John J.; Robertson, Edmund F., "Abu Rayhan Muhammad ibn Ahmad al-Biruni", MacTutor History of Mathematics archive, University of St Andrews.
- ↑ Livingston, John W. (1971). "Ibn Qayyim al-Jawziyyah: A Fourteenth Century Defense against Astrological Divination and Alchemical Transmutation". Journal of the American Oriental Society. American Oriental Society. 91 (1): 96–103 [99]. doi:10.2307/600445. JSTOR 600445.
- ↑ Ragep, Jamil (1993). Nasir al-Din al-Tusi’s Memoir on Astronomy (al-Tadhkira fi `ilm al-hay’ a). New York: Springer-Verlag. p. 129.
- ↑ Galileo Galilei, Sidereus Nuncius (Venice, (Italy): Thomas Baglioni, 1610), pages 15 and 16.
English translation: Galileo Galilei with Edward Stafford Carlos, trans., The Sidereal Messenger (London, England: Rivingtons, 1880), pages 42 and 43. - ↑ O'Connor, J. J.; Robertson, E. F. (November 2002). "Galileo Galilei". University of St. Andrews. Retrieved January 8, 2007.
- ↑ Thomas Wright, An Original Theory or New Hypothesis of the Universe … (London, England: H. Chapelle, 1750).
- On page 57, Wright stated that despite their mutual gravitational attraction, the stars in the constellations don't collide because they are in orbit, so centrifugal force keeps them separated: " … centrifugal force, which not only preserves them in their orbits, but prevents them from rushing all together, by the common universal law of gravity, … "
- On page 48, Wright stated that the form of the Milky Way is a ring: " … the stars are not infinitely dispersed and distributed in a promiscuous manner throughout all the mundane space, without order or design, … this phænomenon [is] no other than a certain effect arising from the observer's situation, … To a spectator placed in an indefinite space, … it [i.e. the Milky Way (Via Lactea)] [is] a vast ring of stars … "
- On page 65, Wright speculated that the central body of the Milky Way, around which the rest of the galaxy revolves, might not be visible to us: " ... the central body A, being supposed as incognitum [i.e. an unknown], without [i.e. outside of] the finite view; ... "
- On page 73, Wright called the Milky Way the Vortex Magnus (the great whirlpool) and estimated its diameter to be 8.64×1012 miles (13.9×1012 km).
- On page 33, Wright speculated that there are a vast number of inhabited planets in the galaxy: " … ; therefore we may justly suppose, that so many radiant bodies [i.e. stars] were not created barely to enlighten an infinite void, but to … display an infinite shapeless universe, crowded with myriads of glorious worlds, all variously revolving round them; and … with an inconceivable variety of beings and states, animate … "
- ↑ Immanuel Kant, Allgemeine Naturgeschichte und Theorie des Himmels … [Universal Natural History and Theory of Heaven … ], (Koenigsberg and Leipzig, (Germany): Johann Friederich Petersen, 1755). On pages 2–3, Kant acknowledged his debt to Thomas Wright: "Dem Herrn Wright von Durham, einen Engeländer, war es vorbehalten, einen glücklichen Schritt zu einer Bemerkung zu thun, welche von ihm selber zu keiner gar zu tüchtigen Absicht gebraucht zu seyn scheinet, und deren nützliche Anwendung er nicht genugsam beobachtet hat. Er betrachtete die Fixsterne nicht als ein ungeordnetes und ohne Absicht zerstreutes Gewimmel, sondern er fand eine systematische Verfassung im Ganzen, und eine allgemeine Beziehung dieser Gestirne gegen einen Hauptplan der Raume, die sie einnehmen." (To Mr. Wright of Durham, an Englishman, it was reserved to take a happy step towards an observation, which seemed, to him and to no one else, to be needed for a clever idea, the exploitation of which he hasn't studied sufficiently. He regarded the fixed stars not as a disorganized swarm that was scattered without a design; rather, he found a systematic shape in the whole, and a general relation between these stars and the principal plane of the space that they occupy.)
- ↑ Kant (1755), pages xxxiii–xxxvi of the Preface (Vorrede): "Ich betrachtete die Art neblichter Sterne, deren Herr von Maupertuis in der Abhandlung von der Figur der Gestirne gedenket, und die die Figur von mehr oder weniger offenen Ellipsen vorstellen, und versicherte mich leicht, daß sie nichts anders als eine Häufung vieler Fixsterne seyn können. Die jederzeit abgemessene Rundung dieser Figuren belehrte mich, daß hier ein unbegreiflich zahlreiches Sternenheer, und zwar um einen gemeinschaftlichen Mittelpunkt, müste geordnet seyn, weil sonst ihre freye Stellungen gegen einander, wohl irreguläre Gestalten, aber nicht abgemessene Figuren vorstellen würden. Ich sahe auch ein: daß sie in dem System, darinn sie sich vereinigt befinden, vornemlich auf eine Fläche beschränkt seyn müßten, weil sie nicht zirkelrunde, sondern elliptische Figuren abbilden, und daß sie wegen ihres blassen Lichts unbegreiflich weit von uns abstehen." (I considered the type of nebulous stars, which Mr. de Maupertuis considered in his treatise on the shape of stars, and which present the figures of more or less open ellipses, and I readily assured myself, that they could be nothing else than a cluster of fixed stars. That these figures always measured round informed me that here an inconceivably numerous host of stars, [which were clustered] around a common center, must be orderly, because otherwise their free positions among each other would likely present irregular forms, not measurable figures. I also realized: that in the system in which they find themselves bound, they must be restricted primarily to a plane, because they display not circular, but elliptical figures, and that on account of their faint light, they are located inconceivably far from us.)
- ↑ Evans, J. C. (November 24, 1998). "Our Galaxy". George Mason University. Retrieved January 4, 2007.
- ↑ The term Weltinsel (island universe) appears nowhere in Kant's book of 1755. The term first appeared in 1850, in the third volume of von Humboldt's Kosmos: Alexander von Humboldt, Kosmos … , vol. 3 (Stuttgart & Tübingen, (Germany): J.G. Cotta, 1850), pages 187, 189. From page 187: "Thomas Wright von Durham, Kant, Lambert und zuerst auch William Herschel waren geneigt die Gestalt der Milchstraße und die scheinbare Anhäufung der Sterne in derselben als eine Folge der abgeplatteten Gestalt und ungleichen Dimensionen der Weltinsel (Sternschict) zu betrachten, in welche unser Sonnensystem eingeschlossen ist." (Thomas Wright of Durham, Kant, Lambert and first of all also William Herschel were inclined to regard the shape of the Milky Way and the apparent clustering of stars in it as a consequence of the oblate shape and unequal dimensions of the world island (star stratum), in which our solar system is included.)
In the English translation—Alexander von Humboldt with E.C. Otté, trans., Cosmos … (New York City: Harper & Brothers, 1897), vols. 3–5—see page 147. - ↑ William Herschel (1785) "On the Construction of the Heavens," Philosophical Transactions of the Royal Society of London, 75 : 213–266. Herschel's diagram of the Milky Way appears immediately after the article's last page. See:
- ↑ Abbey, Lenny. "The Earl of Rosse and the Leviathan of Parsontown". The Compleat Amateur Astronomer. Archived from the original on May 19, 2013. Retrieved January 4, 2007.
- ↑ Curtis, H. D. (1988). "Novae in spiral nebulae and the Island Universe Theory". Publications of the Astronomical Society of the Pacific. 100: 6–2. Bibcode:1988PASP..100....6C. doi:10.1086/132128.
- ↑ Weaver, Harold F. "Robert Julius Trumpler". National Academy of Sciences. Retrieved January 5, 2007.
- ↑ Hubble, E. P. (1929). "A spiral nebula as a stellar system, Messier 31". The Astrophysical Journal. 69: 103–158. Bibcode:1929ApJ....69..103H. doi:10.1086/143167.
- ↑ "New Milky Way Map Is a Spectacular Billion-Star Atlas". 2016-09-14. Retrieved 2016-09-15.
- ↑ "Gaia > Gaia DR1". www.cosmos.esa.int. Retrieved 2016-09-15.
Further reading
- Thorsten Dambeck in Sky and Telescope, "Gaia's Mission to the Milky Way", March 2008, p. 36–39.
- Cristina Chiappini, The Formation and Evolution of the Milky Way, American Scientist, November/December 2001, pp. 506–515
External links
![]() |
Wikimedia Commons has media related to Milky Way Galaxy. |
![]() |
Wikiquote has quotations related to: Milky Way |
- Milky Way – 3D Map
- Milky Way – Basic plan map – Includes spiral arms and Orion spur
- Milky Way – IRAS (infrared) survey – wikisky.org
- Milky Way – H-Alpha survey – wikisky.org
- Milky Way – MultiWavelength – Images and VRML models (NASA)
- Milky Way – Panorama (9 billion pixels).
- Milky Way – Animated tour, University of South Wales
- Milky Way – SEDS Messier website
- Milky Way – Infrared Images
- Milky Way Video (02:37) – VISTA IR Telescope Image (October 24, 2012)
- Milky Way Video (06:37) – in RealTime (Oregon; September 17, 2016)
- All-Sky Map – CMB radiation (Planck; one-year survey)