Glycerol-3-phosphate dehydrogenase
Glycerol-3-phosphate dehydrogenase (NAD+) | |||||||||
---|---|---|---|---|---|---|---|---|---|
![]() Crystallographic structure of human glycerol-3-phosphate dehydrogenase 1.[1] | |||||||||
Identifiers | |||||||||
EC number | 1.1.1.8 | ||||||||
CAS number | 9075-65-4 | ||||||||
Databases | |||||||||
IntEnz | IntEnz view | ||||||||
BRENDA | BRENDA entry | ||||||||
ExPASy | NiceZyme view | ||||||||
KEGG | KEGG entry | ||||||||
MetaCyc | metabolic pathway | ||||||||
PRIAM | profile | ||||||||
PDB structures | RCSB PDB PDBe PDBsum | ||||||||
Gene Ontology | AmiGO / EGO | ||||||||
|
Glycerol-3-phosphate dehydrogenase (quinone) | |||||||||
---|---|---|---|---|---|---|---|---|---|
Identifiers | |||||||||
EC number | 1.1.5.3 | ||||||||
CAS number | 9001-49-4 | ||||||||
Databases | |||||||||
IntEnz | IntEnz view | ||||||||
BRENDA | BRENDA entry | ||||||||
ExPASy | NiceZyme view | ||||||||
KEGG | KEGG entry | ||||||||
MetaCyc | metabolic pathway | ||||||||
PRIAM | profile | ||||||||
PDB structures | RCSB PDB PDBe PDBsum | ||||||||
|
NAD-dependent glycerol-3-phosphate dehydrogenase N-terminus | |||||||||
---|---|---|---|---|---|---|---|---|---|
![]() crystal structure of the n-(1-d-carboxylethyl)-l-norvaline dehydrogenase from arthrobacter sp. strain 1c | |||||||||
Identifiers | |||||||||
Symbol | NAD_Gly3P_dh_N | ||||||||
Pfam | PF01210 | ||||||||
Pfam clan | CL0063 | ||||||||
InterPro | IPR011128 | ||||||||
PROSITE | PDOC00740 | ||||||||
SCOP | 1m66 | ||||||||
SUPERFAMILY | 1m66 | ||||||||
|
NAD-dependent glycerol-3-phosphate dehydrogenase C-terminus | |||||||||
---|---|---|---|---|---|---|---|---|---|
![]() structure of glycerol-3-phosphate dehydrogenase from archaeoglobus fulgidus | |||||||||
Identifiers | |||||||||
Symbol | NAD_Gly3P_dh_C | ||||||||
Pfam | PF07479 | ||||||||
Pfam clan | CL0106 | ||||||||
InterPro | IPR006109 | ||||||||
PROSITE | PDOC00740 | ||||||||
SCOP | 1m66 | ||||||||
SUPERFAMILY | 1m66 | ||||||||
|
Glycerol-3-phosphate dehydrogenase (GPDH) is an enzyme that catalyzes the reversible redox conversion of dihydroxyacetone phosphate (aka glycerone phosphate, outdated) to sn-glycerol 3-phosphate.[2]
Glycerol-3-phosphate dehydrogenase serves as a major link between carbohydrate metabolism and lipid metabolism. It is also a major contributor of electrons to the electron transport chain in the mitochondria.
Older terms for glycerol-3-phosphate dehydrogenase include alpha glycerol-3-phosphate dehydrogenase (alphaGPDH) and glycerolphosphate dehydrogenase (GPDH). However, glycerol-3-phosphate dehydrogenase is not the same as glyceraldehyde 3-phosphate dehydrogenase (GAPDH), whose substrate is an aldehyde not an alcohol.
Metabolic function
GPDH plays a major role in lipid biosynthesis. Through the reduction of dihydroxyacetone phosphate into glycerol 3-phosphate, GPDH allows the prompt dephosphorylation of glycerol 3-phosphate into glycerol.[3] Additionally, GPDH is responsible for maintaining the redox potential across the inner mitochondrial membrane in glycolysis.[3]

Reaction
The NAD+/NADH coenzyme couple act as an electron reservoir for metabolic redox reactions, carrying electrons from one reaction to another.[5] Most of these metabolism reactions occur in the mitochondria. To regenerate NAD+ for further use, NADH pools in the cytosol must be reoxidized. Since the mitochondrial inner membrane is impermeable to both NADH and NAD+, these cannot be freely exchanged between the cytosol and mitochondrial matrix.[4]
One way to shuttle this reducing equivalent across the membrane is through the Glycerol-3-phosphate shuttle, which employs the two forms of GPDH:
- Cytosolic GPDH, or GPD1 is located in the mitochondrial inner-membrane space or cytosol, and catalyzes the reduction of dihydroxyacetone phosphate into glycerol-3-phosphate.
- In conjunction, Mitochondrial GPDH, or GPD2 is embedded on the outer surface of the inner mitochondrial membrane, overlooking the cytosol, and catalyzes the oxidation of glycerol-3-phosphate to dihydroxyacetone phosphate.[6]
The reactions catalyzed by cytosolic (soluble) and mitochondrial GPDH are as follows:
![]() Coupled reactions catalyzed by the cytosolic (GPDH-C) and mitochondrial (GPDH-M) forms of glycerol 3-phosphate dehydrogenase.[7] GPDH-C and GPDH-M use NADH and quinol (QH) as an electron donors respectively. GPDH-M in addition uses FAD as a co-factor. |
Variants
There are two forms of GPDH:
Enzyme | Protein | Gene | |||||
---|---|---|---|---|---|---|---|
EC number | Name | Donor / Acceptor | Name | Subcellular location | Abbreviation | Name | Symbol |
1.1.1.8 | glycerol-3-phosphate dehydrogenase | NADH / NAD+ | Glycerol-3-phosphate dehydrogenase [NAD+] | cytoplasmic | GPDH-C | glycerol-3-phosphate dehydrogenase 1 (soluble) | GPD1 |
1.1.5.3 | glycerol-3-phosphate dehydrogenase | quinol / quinone | Glycerol-3-phosphate dehydrogenase | mitochondrial | GPDH-M | glycerol-3-phosphate dehydrogenase 2 (mitochondrial) | GPD2 |
The following human genes encode proteins with GPDH enzymatic activity:
|
|
GPD1
Cytosolic Glycerol-3-phosphate dehydrogenase (GPD1), is an NAD+-dependent enzyme[8] that reduces dihydroxyacetone phosphate to glycerol-3-phosphate. Simultaneously, NADH is oxidized to NAD+ in the following reaction:

As a result, NAD+ is regenerated for further metabolic activity.
GPD1 consists of two subunits,[9] and reacts with dihydroxyacetone phosphate and NAD+ though the following interaction:
Figure 4. The putative active site. The phosphate group of DHAP is half-encircled by the side-chain of Arg269, and interacts with Arg269 and Gly268 directly by hydrogen bonds (not shown). The conserved residues Lys204, Asn205, Asp260 and Thr264 form a stable hydrogen bonding network. The other hydrogen bonding network includes residues Lys120 and Asp260, as well as an ordered water molecule (with a B-factor of 16.4 Å2), which hydrogen bonds to Gly149 and Asn151 (not shown). In these two electrostatic networks, only the ε-NH3+ group of Lys204 is the nearest to the C2 atom of DHAP (3.4 Å).[1]
GPD2
Mitochondrial glycerol-3-phosphate dehydrogenase (GPD2), catalyzes the irreversible oxidation of glycerol-3-phosphate to dihydroxyacetone phosphate and concomitantly transfers two electrons from FAD to the electron transport chain. GPD2 consists of 4 identical subunits.[10]
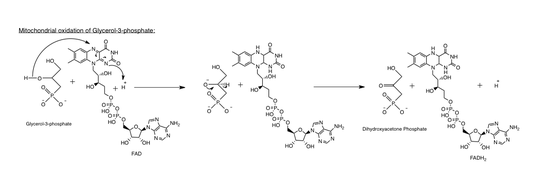
Response to environmental stresses
- Studies indicate that GPDH is mostly unaffected by pH changes: neither GPD1 or GPD2 is favored under certain pH conditions.
- At high salt concentrations (E.g. NaCl), GPD1 activity is enhanced over GPD2, since an increase in the salinity of the medium leads to an accumulation of glycerol in response.
- Changes in temperature do not appear to favor neither GPD1 nor GPD2.[11]
Glycerol-3-phosphate shuttle
The cytosolic together with the mitochondrial glycerol-3-phosphate dehydrogenase work in concert. Oxidation of cytoplasmic NADH by the cytosolic form of the enzyme creates glycerol-3-phosphate from dihydroxyacetone phosphate. Once the glycerol-3-phosphate has moved through the inner mitochondrial membrane it can then be oxidised by a separate isoform of glycerol-3-phosphate dehydrogenase that uses quinone as an oxidant and FAD as a co-factor. As a result, there is a net loss in energy, comparable to one molecule of ATP.[7]
The combined action of these enzymes maintains the NAD+/NADH ratio that allows for continuous operation of metabolism.
Role in disease
The fundamental role of GDPH in maintaining the NAD+/NADH potential, as well as its role in lipid metabolism, makes GDPH a factor in lipid imbalance diseases, such as obesity.
- Enhanced GPDH activity, particularly GPD2, leads to an increase in glycerol production. Since glycerol is a main subunit in lipid metabolism, its abundance can easily lead to an increase in triglyceride accumulation at a cellular level. As a result, there is a tendency to form adipose tissue leading to an accumulation of fat that favors obesity.[12]
- GPDH has also been found to play a role in Brugada syndrome. Mutations in the gene encoding GPD1 have been proven to cause defects in the electron transport chain. This conflict with NAD+/NADH levels in the cell is believed to contribute to defects in cardiac sodium ion channel regulation and can lead to a lethal arrythmia during infancy.[13]
Pharmacological target
The mitochondrial isoform of G3P dehydrogenase is thought to be inhibited by metformin, a first line drug for type 2 diabetes. [14]
Structure
Glycerol-3-phosphate dehydrogenase consists of two protein domains. The N-terminal domain is an NAD-binding domain, and the C-terminus acts as a substrate-binding domain.[15] However, dimer and tetramer interface residues are involved in GAPDH-RNA binding, as GAPDH can exhibit several moonlighting activities, including the modulation of RNA binding and/or stability.[16]
See also
- substrate pages: glycerol 3-phosphate, dihydroxyacetone phosphate
- related topics: glycerol phosphate shuttle, creatine kinase, glycolysis, gluconeogenesis
References
- 1 2 PDB: 1X0V; Ou X, Ji C, Han X, Zhao X, Li X, Mao Y, Wong LL, Bartlam M, Rao Z (Mar 2006). "Crystal structures of human glycerol 3-phosphate dehydrogenase 1 (GPD1)". Journal of Molecular Biology. 357 (3): 858–69. doi:10.1016/j.jmb.2005.12.074. PMID 16460752.
- ↑ Ou X, Ji C, Han X, Zhao X, Li X, Mao Y, Wong LL, Bartlam M, Rao Z (Mar 2006). "Crystal structures of human glycerol 3-phosphate dehydrogenase 1 (GPD1)". Journal of Molecular Biology. 357 (3): 858–69. doi:10.1016/j.jmb.2005.12.074. PMID 16460752.
- 1 2 Harding JW, Pyeritz EA, Copeland ES, White HB (Jan 1975). "Role of glycerol 3-phosphate dehydrogenase in glyceride metabolism. Effect of diet on enzyme activities in chicken liver" (PDF). The Biochemical Journal. 146 (1): 223–9. doi:10.1042/bj1460223. PMC 1165291
. PMID 167714.
- 1 2 Geertman JM, van Maris AJ, van Dijken JP, Pronk JT (Nov 2006). "Physiological and genetic engineering of cytosolic redox metabolism in Saccharomyces cerevisiae for improved glycerol production". Metabolic Engineering. 8 (6): 532–42. doi:10.1016/j.ymben.2006.06.004. PMID 16891140.
- ↑ Ansell R, Granath K, Hohmann S, Thevelein JM, Adler L (May 1997). "The two isoenzymes for yeast NAD+-dependent glycerol 3-phosphate dehydrogenase encoded by GPD1 and GPD2 have distinct roles in osmoadaptation and redox regulation". The EMBO Journal. 16 (9): 2179–87. doi:10.1093/emboj/16.9.2179. PMC 1169820
. PMID 9171333.
- ↑ Kota V, Rai P, Weitzel JM, Middendorff R, Bhande SS, Shivaji S (Sep 2010). "Role of glycerol-3-phosphate dehydrogenase 2 in mouse sperm capacitation". Molecular Reproduction and Development. 77 (9): 773–83. doi:10.1002/mrd.21218. PMID 20602492.
- 1 2 Stryer, Lubert; Berg, Jeremy Mark; Tymoczko, John L. (2002). "Chapter 18.5: Glycerol 3-Phosphate Shuttle". Biochemistry. San Francisco: W.H. Freeman. ISBN 0-7167-4684-0.
- ↑ Guindalini C, Lee KS, Andersen ML, Santos-Silva R, Bittencourt LR, Tufik S (Jan 2010). "The influence of obstructive sleep apnea on the expression of glycerol-3-phosphate dehydrogenase 1 gene". Experimental Biology and Medicine. 235 (1): 52–6. doi:10.1258/ebm.2009.009150. PMID 20404019.
- ↑ Bunoust O, Devin A, Avéret N, Camougrand N, Rigoulet M (Feb 2005). "Competition of electrons to enter the respiratory chain: a new regulatory mechanism of oxidative metabolism in Saccharomyces cerevisiae". The Journal of Biological Chemistry. 280 (5): 3407–13. doi:10.1074/jbc.M407746200. PMID 15557339.
- ↑ Kota V, Dhople VM, Shivaji S (Apr 2009). "Tyrosine phosphoproteome of hamster spermatozoa: role of glycerol-3-phosphate dehydrogenase 2 in sperm capacitation". Proteomics. 9 (7): 1809–26. doi:10.1002/pmic.200800519. PMID 19333995.
- ↑ Kumar S, Kalyanasundaram GT, Gummadi SN (Feb 2011). "Differential response of the catalase, superoxide dismutase and glycerol-3-phosphate dehydrogenase to different environmental stresses in Debaryomyces nepalensis NCYC 3413". Current Microbiology. 62 (2): 382–7. doi:10.1007/s00284-010-9717-z. PMID 20644932.
- ↑ Xu SP, Mao XY, Ren FZ, Che HL (Feb 2011). "Attenuating effect of casein glycomacropeptide on proliferation, differentiation, and lipid accumulation of in vitro Sprague-Dawley rat preadipocytes". Journal of Dairy Science. 94 (2): 676–83. doi:10.3168/jds.2010-3827. PMID 21257036.
- ↑ Van Norstrand DW, Valdivia CR, Tester DJ, Ueda K, London B, Makielski JC, Ackerman MJ (Nov 2007). "Molecular and functional characterization of novel glycerol-3-phosphate dehydrogenase 1 like gene (GPD1-L) mutations in sudden infant death syndrome". Circulation. 116 (20): 2253–9. doi:10.1161/CIRCULATIONAHA.107.704627. PMC 3332545
. PMID 17967976.
- ↑ Ferrannini E (Oct 2014). "The target of metformin in type 2 diabetes". The New England Journal of Medicine. 371 (16): 1547–8. doi:10.1056/NEJMcibr1409796. PMID 25317875.
- ↑ Suresh S, Turley S, Opperdoes FR, Michels PA, Hol WG (May 2000). "A potential target enzyme for trypanocidal drugs revealed by the crystal structure of NAD-dependent glycerol-3-phosphate dehydrogenase from Leishmania mexicana". Structure. 8 (5): 541–52. doi:10.1016/s0969-2126(00)00135-0. PMID 10801498.
- ↑ White MR, Khan MM, Deredge D, Ross CR, Quintyn R, Zucconi BE, Wysocki VH, Wintrode PL, Wilson GM, Garcin ED (Jan 2015). "A dimer interface mutation in glyceraldehyde-3-phosphate dehydrogenase regulates its binding to AU-rich RNA". The Journal of Biological Chemistry. 290 (3): 1770–85. doi:10.1074/jbc.M114.618165. PMC 4340419
. PMID 25451934.
Further reading
- Baranowski T (1963). "α-Glycerophosphate dehydrogenase". In Boyer PD, Lardy H, Myrbäck K. The Enzymes (2nd ed.). New York: Academic Press. pp. 85–96.
- Brosemer RW, Kuhn RW (May 1969). "Comparative structural properties of honeybee and rabbit alpha-glycerophosphate dehydrogenases". Biochemistry. 8 (5): 2095–105. doi:10.1021/bi00833a047. PMID 4307630.
- O'Brien SJ, MacIntyre RJ (Oct 1972). "The -glycerophosphate cycle in Drosophila melanogaster. I. Biochemical and developmental aspects". Biochemical Genetics. 7 (2): 141–61. doi:10.1007/BF00486085. PMID 4340553.
- Warkentin DL, Fondy TP (Jul 1973). "Isolation and characterization of cytoplasmic L-glycerol-3-phosphate dehydrogenase from rabbit-renal-adipose tissue and its comparison with the skeletal-muscle enzyme". European Journal of Biochemistry / FEBS. 36 (1): 97–109. doi:10.1111/j.1432-1033.1973.tb02889.x. PMID 4200180.
- Albertyn J, van Tonder A, Prior BA (Aug 1992). "Purification and characterization of glycerol-3-phosphate dehydrogenase of Saccharomyces cerevisiae". FEBS Letters. 308 (2): 130–2. doi:10.1016/0014-5793(92)81259-O. PMID 1499720.
- Koekemoer TC, Litthauer D, Oelofsen W (Jun 1995). "Isolation and characterization of adipose tissue glycerol-3-phosphate dehydrogenase". The International Journal of Biochemistry & Cell Biology. 27 (6): 625–32. doi:10.1016/1357-2725(95)00012-E. PMID 7671141.
- Påhlman IL, Larsson C, Averét N, Bunoust O, Boubekeur S, Gustafsson L, Rigoulet M (Aug 2002). "Kinetic regulation of the mitochondrial glycerol-3-phosphate dehydrogenase by the external NADH dehydrogenase in Saccharomyces cerevisiae". The Journal of Biological Chemistry. 277 (31): 27991–5. doi:10.1074/jbc.M204079200. PMID 12032156.
- Overkamp KM, Bakker BM, Kötter P, van Tuijl A, de Vries S, van Dijken JP, Pronk JT (May 2000). "In vivo analysis of the mechanisms for oxidation of cytosolic NADH by Saccharomyces cerevisiae mitochondria". Journal of Bacteriology. 182 (10): 2823–30. doi:10.1128/JB.182.10.2823-2830.2000. PMC 101991
. PMID 10781551.
- Dawson AG, Cooney GJ (Jul 1978). "Reconstruction of the alpha-glycerolphosphate shuttle using rat kidney mitochondria". FEBS Letters. 91 (2): 169–72. doi:10.1016/0014-5793(78)81164-8. PMID 210038.
- Opperdoes FR, Borst P, Bakker S, Leene W (Jun 1977). "Localization of glycerol-3-phosphate oxidase in the mitochondrion and particulate NAD+-linked glycerol-3-phosphate dehydrogenase in the microbodies of the bloodstream form to Trypanosoma brucei". European Journal of Biochemistry / FEBS. 76 (1): 29–39. doi:10.1111/j.1432-1033.1977.tb11567.x. PMID 142010.
- Eswaramoorthy S, Bonanno JB, Burley SK, Swaminathan S (Jun 2006). "Mechanism of action of a flavin-containing monooxygenase". Proceedings of the National Academy of Sciences of the United States of America. 103 (26): 9832–7. doi:10.1073/pnas.0602398103. PMC 1502539
. PMID 16777962.
External links
- equivalent entries:
- alphaGPDH at the US National Library of Medicine Medical Subject Headings (MeSH)
- GPDH
- Yeast genome database GO term: GPDH
- enzyme no. -2053504966 at GPnotebook
This article incorporates text from the public domain Pfam and InterPro IPR011128
This article incorporates text from the public domain Pfam and InterPro IPR006109