Aeolis quadrangle
Map of Aeolis quadrangle from Mars Orbiter Laser Altimeter (MOLA) data. The highest elevations are red and the lowest are blue. The Spirit rover landed in Gusev crater. Aeolis Mons is in Gale Crater. | |
Coordinates | 15°00′S 202°30′W / 15°S 202.5°WCoordinates: 15°00′S 202°30′W / 15°S 202.5°W |
---|

The Aeolis quadrangle is one of a series of 30 quadrangle maps of Mars used by the United States Geological Survey (USGS) Astrogeology Research Program. The Aeolis quadrangle is also referred to as MC-23 (Mars Chart-23).[1] The Aeolis quadrangle covers 180° to 225° W and 0° to 30° south on Mars, and contains parts of the regions Elysium Planitia and Terra Cimmeria. A small part of the Medusae Fossae Formation lies in this quadrangle.
It is famous as the site of two spacecraft landings: the Spirit Rover landing site (14°34′18″S 175°28′43″E / 14.5718°S 175.4785°E) in Gusev crater (January 4, 2004), and the Curiosity Rover in Gale Crater (4°35′31″S 137°26′25″E / 4.591817°S 137.440247°E) (August 6, 2012).[2]
A large, ancient river valley, called Ma'adim Vallis, enters at the south rim of Gusev Crater, so Gusev Crater was believed to be an ancient lake bed. However, it seems that a volcanic flow covered up the lakebed sediments.[3] Apollinaris Patera, a large volcano, lies directly north of Gusev Crater.[4]
Gale Crater, in the northwestern part of the Aeolis quadrangle, is of special interest to geologists because it contains a 2–4 km (1.2–2.5 mile) high mound of layered sedimentary rocks, named "Mount Sharp" by NASA in honor of Robert P. Sharp (1911–2004), a planetary scientist of early Mars missions.[5][6][7] More recently, on 16 May 2012, "Mount Sharp" was officially named Aeolis Mons by the USGS and IAU.[8]
Some regions in the Aeolis quadrangle show inverted relief.[9] In these locations, a stream bed may be a raised feature, instead of a valley. The inverted former stream channels may be caused by the deposition of large rocks or due to cementation. In either case erosion would erode the surrounding land but leave the old channel as a raised ridge because the ridge will be more resistant to erosion
Yardangs are another feature found in this quadrangle They are generally visible as a series of parallel linear ridges, caused by the direction of the prevailing wind.
Spirit Rover discoveries
The rocks on the plains of Gusev are a type of basalt. They contain the minerals olivine, pyroxene, plagioclase, and magnetite, and they look like volcanic basalt as they are fine-grained with irregular holes (geologists would say they have vesicles and vugs).[10][11] Much of the soil on the plains came from the breakdown of the local rocks. Fairly high levels of nickel were found in some soils; probably from meteorites.[12] Analysis shows that the rocks have been slightly altered by tiny amounts of water. Outside coatings and cracks inside the rocks suggest water deposited minerals, maybe bromine compounds. All the rocks contain a fine coating of dust and one or more harder kinds of material. One type can be brushed off, while another needed to be ground off by the Rock Abrasion Tool (RAT).[13]
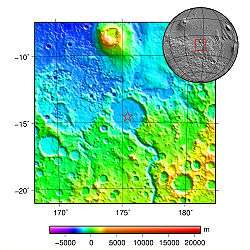
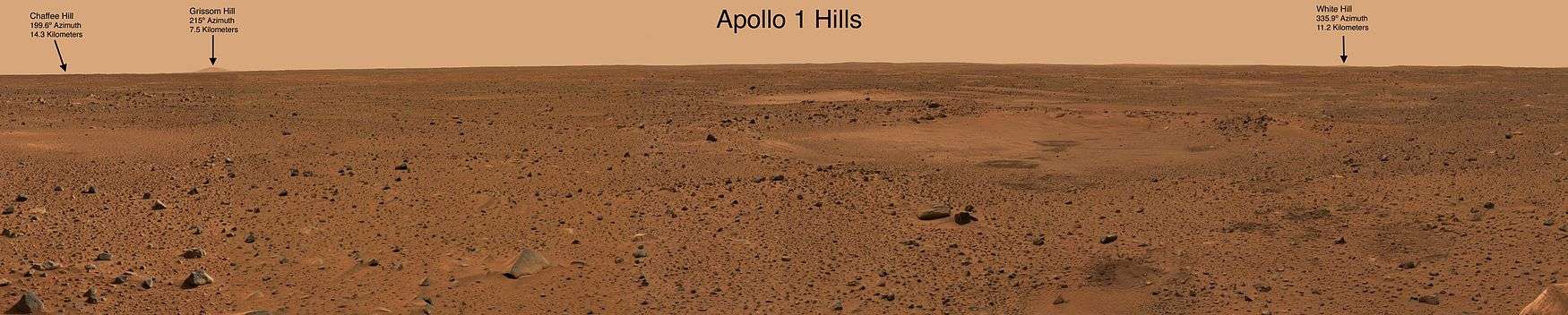
There are a variety of rocks in the Columbia Hills (Mars), some of which have been altered by water, but not by very much water.
The dust in Gusev Crater is the same as dust all around the planet. All the dust was found to be magnetic. Moreover, Spirit found the magnetism was caused by the mineral magnetite, especially magnetite that contained the element titanium. One magnet was able to completely divert all dust hence all Martian dust is thought to be magnetic.[14] The spectra of the dust was similar to spectra of bright, low thermal inertia regions like Tharsis and Arabia that have been detected by orbiting satellites. A thin layer of dust, maybe less than one millimeter thick covers all surfaces. Something in it contains a small amount of chemically bound water.[15][16]
Plains
![]() Above: An approximate true-color view of Adirondack, taken by Spirit's pancam. Right:Digital camera image (from Spirit's Pancam) of Adirondack after a RAT grind (Spirit's rock grinding tool) | |
Feature type | Rock |
---|
Observations of rocks on the plains show they contain the minerals pyroxene, olivine, plagioclase, and magnetite. These rocks can be classified in different ways. The amounts and types of minerals make the rocks primitive basalts—also called picritic basalts. The rocks are similar to ancient terrestrial rocks called basaltic komatiites. Rocks of the plains also resemble the basaltic shergottites, meteorites which came from Mars. One classification system compares the amount of alkali elements to the amount of silica on a graph; in this system, Gusev plains rocks lie near the junction of basalt, picrobasalt, and tephite. The Irvine-Barager classification calls them basalts.[17] Plain’s rocks have been very slightly altered, probably by thin films of water because they are softer and contain veins of light colored material that may be bromine compounds, as well as coatings or rinds. It is thought that small amounts of water may have gotten into cracks inducing mineralization processes.[11][17] Coatings on the rocks may have occurred when rocks were buried and interacted with thin films of water and dust. One sign that they were altered was that it was easier to grind these rocks compared to the same types of rocks found on Earth.
The first rock that Spirit studied was Adirondack. It turned out to be typical of the other rocks on the plains.
-
First color picture from Gusev crater. Rocks were found to be basalt. Everything was covered with a fine dust that Spirit determined was magnetic because of the mineral magnetite.
-
Cross-sectional drawing of a typical rock from the plains of Gusev crater. Most rocks contain a coating of dust and one or more harder coatings. Veins of water-deposited veins are visible, along with crystals of olivine. Veins may contain bromine salts.
Columbia Hills
Scientists found a variety of rock types in the Columbia Hills, and they placed them into six different categories. The six are: Clovis, Wishbone, Peace, Watchtower, Backstay, and Independence. They are named after a prominent rock in each group. Their chemical compositions, as measured by APXS, are significantly different from each other.[18] Most importantly, all of the rocks in Columbia Hills show various degrees of alteration due to aqueous fluids.[19] They are enriched in the elements phosphorus, sulfur, chlorine, and bromine—all of which can be carried around in water solutions. The Columbia Hills’ rocks contain basaltic glass, along with varying amounts of olivine and sulfates.[20][21] The olivine abundance varies inversely with the amount of sulfates. This is exactly what is expected because water destroys olivine but helps to produce sulfates.
Acid fog is believed to have changed some of the Watchtower rocks. This was in a 200 meter long section of Cumberland Ridge and the Husband Hill summit. Certain places became less crystalline and more amorphous. Acidic water vapor from volcanoes dissolved some minerals forming a gel. When water evaporated a cement formed and produced small bumps. This type of process has been observed in the lab when basalt rocks are exposed to sulfuric and hydrochloric acids.[22][23][24]
The Clovis group is especially interesting because the Mössbauer spectrometer(MB) detected goethite in it.[25] Goethite forms only in the presence of water, so its discovery is the first direct evidence of past water in the Columbia Hills's rocks. In addition, the MB spectra of rocks and outcrops displayed a strong decline in olivine presence,[20] although the rocks probably once contained much olivine.[26] Olivine is a marker for the lack of water because it easily decomposes in the presence of water. Sulfate was found, and it needs water to form. Wishstone contained a great deal of plagioclase, some olivine, and anhydrate (a sulfate). Peace rocks showed sulfur and strong evidence for bound water, so hydrated sulfates are suspected. Watchtower class rocks lack olivine consequently they may have been altered by water. The Independence class showed some signs of clay (perhaps montmorillonite a member of the smectite group). Clays require fairly long term exposure to water to form. One type of soil, called Paso Robles, from the Columbia Hills, may be an evaporate deposit because it contains large amounts of sulfur, phosphorus, calcium, and iron.[27] Also, MB found that much of the iron in Paso Robles soil was of the oxidized, Fe+++ form, which would happen if water had been present.[15]
Towards the middle of the six-year mission (a mission that was supposed to last only 90 days), large amounts of pure silica were found in the soil. The silica could have come from the interaction of soil with acid vapors produced by volcanic activity in the presence of water or from water in a hot spring environment.[28]
After Spirit stopped working scientists studied old data from the Miniature Thermal Emission Spectrometer, or Mini-TES and confirmed the presence of large amounts of carbonate-rich rocks, which means that regions of the planet may have once harbored water. The carbonates were discovered in an outcrop of rocks called "Comanche."[29][30]
In summary, Spirit found evidence of slight weathering on the plains of Gusev, but no evidence that a lake was there. However, in the Columbia Hills there was clear evidence for a moderate amount of aqueous weathering. The evidence included sulfates and the minerals goethite and carbonates which only form in the presence of water. It is believed that Gusev crater may have held a lake long ago, but it has since been covered by igneous materials. All the dust contains a magnetic component which was identified as magnetite with some titanium. Furthermore, the thin coating of dust that covers everything on Mars is the same in all parts of Mars.
Ma'adim Vallis
A large, ancient river valley, called Ma'adim Vallis, enters at the south rim of Gusev Crater, so Gusev Crater was believed to be an ancient lake bed. However, it seems that a volcanic flow covered up the lakebed sediments.[3] Apollinaris Patera, a large volcano, lies directly north of Gusev Crater.[4]
Recent studies lead scientists to believe that the water that formed Ma'adim Vallis originated in a complex of lakes.[31][32][33] The largest lake is located at the source of the Ma'adim Vallis outflow channel and extends into Eridania quadrangle and the Phaethontis quadrangle.[34] When the largest lake spilled over the low point in its boundary, a torrential flood would have moved north, carving the sinuous Ma'adim Vallis. At the north end of Ma'adim Vallis, the flood waters would have run into Gusev Crater.[35]
There is enormous evidence that water once flowed in river valleys on Mars. Images of curved channels have been seen in images from Mars spacecraft dating back to the early seventies with the Mariner 9 orbiter.[36][37][38][39] Vallis (plural valles) is the Latin word for valley. It is used in planetary geology for the naming of landform features on other planets, including what could be old river valleys that were discovered on Mars, when probes were first sent to Mars. The Viking Orbiters caused a revolution in our ideas about water on Mars; huge river valleys were found in many areas. Space craft cameras showed that floods of water broke through dams, carved deep valleys, eroded grooves into bedrock, and traveled thousands of kilometers.[40][41][42] Some valles on Mars (Mangala Vallis, Athabasca Vallis, Granicus Vallis, and Tinjar Valles) clearly begin at graben. On the other hand, some of the large outflow channels begin in rubble-filled low areas called chaos or chaotic terrain. It has been suggested that massive amounts of water were trapped under pressure beneath a thick cryosphere (layer of frozen ground), then the water was suddenly released, perhaps when the cryosphere was broken by a fault.[43][44]
-
Section of Ma'adim Vallis as seen by HiRISE. A more recent flow of water may have formed the smaller, deeper channel to the right.
Gale Crater
Gale Crater, in the northwestern part of the Aeolis quadrangle, is of special interest to geologists because it contains a 2–4 km (1.2–2.5 mile) high mound of layered sedimentary rocks. On 28 March 2012 this mound was named "Mount Sharp" by NASA in honor of Robert P. Sharp (1911–2004), a planetary scientist of early Mars missions.[5][6][7] More recently, on 16 May 2012, Mount Sharp was officially named Aeolis Mons by the USGS and IAU.[8] The mound extends higher than the rim of the crater, so perhaps the layering covered an area much larger than the crater.[45] These layers are a complex record of the past. The rock layers probably took millions of years to be laid down within the crater, then more time to be eroded to make them visible.[46] The 5 km high mound is probably the thickest single succession of sedimentary rocks on Mars.[47] The lower formation may date from near the Noachian age, while the upper layer, separated by an erosional unconformity, may be as young as the Amazonian period.[48] The lower formation may have formed the same time as parts of Sinus Meridiani and Mawrth Vallis. The upper layer may be similar to layers in Arabia Terra. Sulfates and Iron oxides have been detected in the lower formation and anhydrous phases in the upper layer.[49] There is evidence that the first phase of erosion was followed by more cratering and more rock formation.[50] Also of interest in Gale Crater is Peace Vallis, officially named by the IAU on September 26, 2012,[51] which 'flows' down out of the Gale Crater hills to the Aeolis Palus below, and which seems to have been carved by flowing water.[52][53][54] On December 9, 2013, NASA reported that, based on evidence from Curiosity studying Aeolis Palus, Gale Crater contained an ancient freshwater lake which could have been a hospitable environment for microbial life.[55][56] Gale Crater contains a number of fans and deltas that provide information about lake levels in the past. These formations are: Pancake Delta, Western Delta, Farah Vallis delta and the Peace Vallis Fan.[57]
-
Gale crater - surface materials (false colors; THEMIS; 2001 Mars Odyssey).
-
Gale Crater with Aeolis Mons rising from the center. The noted Curiosity Rover landing area is near Peace Vallis in Aeolis Palus.
-
Aeolis Mons rises from the middle of Gale Crater - Green dot marks the Curiosity Rover landing site in Aeolis Palus.
-
Ancient Lake fills Gale Crater on Mars (simulated view).
-
Ancient Lake on Aeolis Palus in Gale Crater - possible size (December 9, 2013).[1][2]
-
Peace Vallis and alluvial fan near the Curiosity rover landing ellipse and site (noted by +).
- ^ Cite error: The named reference
NYT-20131209
was invoked but never defined (see the help page). - ^ Cite error: The named reference
SCI-20131209
was invoked but never defined (see the help page).
-
Curiosity Rover landing site (green dot) - Blue dot marks Glenelg Intrigue - Blue spot marks "Base of Mount Sharp" - a planned area of study.
-
Curiosity Rover landing site - "Quad Map" includes "Yellowknife" Quad 51 of Aeolis Palus in Gale Crater.
-
Gale Crater Grand Canyon, as seen by HiRISE - Scale bar is 500 meters long.
-
Gale Crater sediment layers may have formed by lake or windblown particle deposition.
-
Gale Crater rim about 18 km (11 mi) North of the Curiosity Rover on August 9, 2012.
-
Aeolis Mons and Aeolis Palus in Gale Crater as viewed by the Curiosity Rover on August 6, 2012.
-
Aeolis Mons may have formed from the erosion of sediment layers that once filled Gale Crater.
-
Aeolis Mons as viewed from the Curiosity Rover (August 9, 2012) (white balanced image).
-
Layers at the base of Aeolis Mons - dark rock in inset is same size as the Curiosity Rover (white balanced image).
-
Wheels on the Curiosity Rover - "Mount Sharp" is in the background (MAHLI, September 9, 2012).
-
First-Year & First-Mile Traverse Map of the Curiosity rover on Mars (August 1, 2013) (3-D).



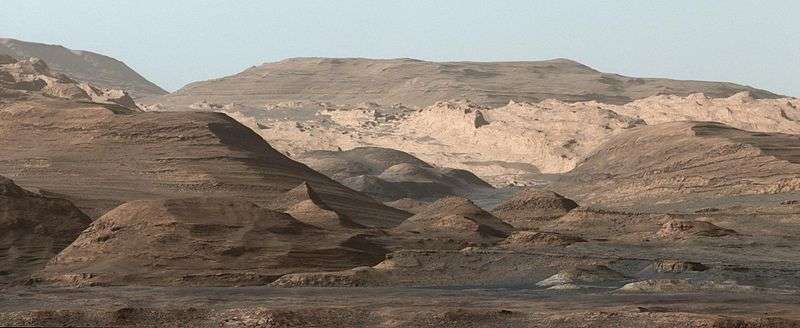
Other craters
Impact craters generally have a rim with ejecta around them, in contrast volcanic craters usually do not have a rim or ejecta deposits. As craters get larger (greater than 10 km in diameter) they usually have a central peak.[58] The peak is caused by a rebound of the crater floor following the impact.[40] Sometimes craters will display layers. Since the collision that produces a crater is like a powerful explosion, rocks from deep underground are tossed onto the surface. Hence, craters can show us what lies deep under the surface.
-
Boeddicker Crater Floor, as seen by HiRISE.
-
Central uplift of an Unnamed crater on the floor of Molesworth Crater, as seen by HiRISE. Dark sand dunes are on left side of image. The scale bar is 500 meters long.
-
Reuyl Crater Central Peak, as seen by HiRISE.
-
Galdakao Crater,as seen by HiRISE. Click on image to see Dark Slope Streaks.
-
Layers in crater wall, as seen by HiRISE under HiWish program. Area in box is enlarged in the next image.
-
Enlargement from previous image, showing many thin layers. Note that the layers do not seem to be formed from rocks. They may be all that is left of a deposit that once filled the crater. Image was taken with HiRISE, under HiWish program.
-
Gullies on wall of impact crater, as seen by HiRISE under HiWish program. Curved ridges on the floor are remains of old glaciers.
-
Graff Crater (Martian Crater), as seen by CTX camera (on Mars Reconnaissance Orbiter).
Mars Science Laboratory discoveries
The aim of the Mars Science Laboratory mission, and its surface robotic payload Curiosity rover, is to search for signs of ancient life. It is hoped that a later mission could then return samples that the laboratory identified as probably containing remains of life. To safely bring the craft down, a 12 mile wide, smooth, flat circle was needed. Geologists hoped to examine places where water once ponded[59] and to examine sedimentary layers.
On August 6, 2012, the Mars Science Laboratory landed on Aeolis Palus near Aeolis Mons in Gale Crater.[5][6][7][8] [60] [61] The landing was 2.279 km (1.416 mi) from the target (4°35′31″S 137°26′25″E / 4.591817°S 137.440247°E), closer than any previous rover landing and well within the target area.
On September 27, 2012, NASA scientists announced that the Curiosity rover found evidence for an ancient streambed suggesting a "vigorous flow" of water on Mars.[52][53][54]
On October 17, 2012, at Rocknest, the first X-ray diffraction analysis of Martian soil was performed. The results revealed the presence of several minerals, including feldspar, pyroxenes and olivine, and suggested that the Martian soil in the sample was similar to the weathered basaltic soils of Hawaiian volcanoes. The sample used is composed of dust distributed from global dust storms and local fine sand. So far, the materials Curiosity has analyzed are consistent with the initial ideas of deposits in Gale Crater recording a transition through time from a wet to dry environment.[64]
On December 3, 2012, NASA reported that Curiosity performed its first extensive soil analysis, revealing the presence of water molecules, sulfur and chlorine in the Martian soil.[65][66] The presence of perchlorates in the sample seems highly likely. The presence of sulfate and sulfide is also likely because sulfur dioxide and hydrogen sulfide were detected. Small amounts of chloromethane, dichloromethane and trichloromethane were detected. The source of the carbon in these molecules is unclear. Possible sources include contamination of the instrument, organics in the sample and inorganic carbonates.[65][66]
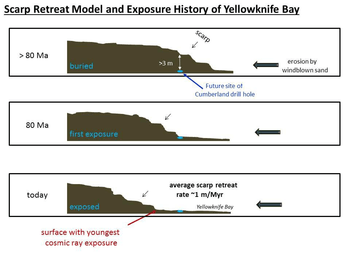
On March 18, 2013, NASA reported evidence of mineral hydration, likely hydrated calcium sulfate, in several rock samples including the broken fragments of "Tintina" rock and "Sutton Inlier" rock as well as in veins and nodules in other rocks like "Knorr" rock and "Wernicke" rock.[67][68][69] Analysis using the rover's DAN instrument provided evidence of subsurface water, amounting to as much as 4% water content, down to a depth of 60 cm (2.0 ft), in the rover's traverse from the Bradbury Landing site to the Yellowknife Bay area in the Glenelg terrain.[67]
In March 2013, NASA reported Curiosity found evidence that geochemical conditions in Gale Crater were once suitable for microbial life after analyzing the first drilled sample of Martian rock, "John Klein" rock at Yellowknife Bay in Gale Crater. The rover detected water, carbon dioxide, oxygen, sulfur dioxide and hydrogen sulfide.[70][71][72] Chloromethane and dichloromethane were also detected. Related tests found results consistent with the presence of smectite clay minerals.[70][71][72][73][74]
In the journal Science from September 2013, researchers described a different type of rock called "Jake M" or "Jake Matijevic (rock),” It was the first rock analyzed by the Alpha Particle X-ray Spectrometer instrument on the Curiosity rover, and it was different from other known martian igneous rocks as it is alkaline (>15% normative nepheline) and relatively fractionated. Jake M is similar to terrestrial mugearites, a rock type typically found at ocean islands and continental rifts. Jake M's discovery may mean that alkaline magmas may be more common on Mars than on Earth and that Curiosity could encounter even more fractionated alkaline rocks (for example, phonolites and trachytes).[75]

On December 9, 2013, NASA researchers described, in a series of six articles in the journal Science, many new discoveries from the Curiosity rover. Possible organics were found that could not be explained by contamination.[76][77] Although the organic carbon was probably from Mars, it can all be explained by dust and meteorites that have landed on the planet.[78][79][80] Because much of the carbon was released at a relatively low temperature in Curiosity’s Sample Analysis at Mars (SAM) instrument package, it probably did not come from carbonates in the sample. The carbon could be from organisms, but this has not been proven. This organic-bearing material was obtained by drilling 5 centimeters deep in a site called Yellowknife Bay into a rock called “Sheepbed mudstone”. The samples were named John Klein and Cumberland. Microbes could be living on Mars by obtaining energy from chemical imbalances between minerals in a process called chemolithotrophy which means “eating rock.”[81] However, in this process only a very tiny amount of carbon is involved — much less than was found at Yellowknife Bay.[82][83]
Using SAM’s mass spectrometer, scientists measured isotopes of helium, neon, and argon that cosmic rays produce as they go through rock. The fewer of these isotopes they find, the more recently the rock has been exposed near the surface. The 4-billion-year-old lakebed rock drilled by Curiosity was uncovered between 30 million and 110 million years ago by winds which sandblasted away 2 meters of overlying rock. Next, they hope to find a site tens of millions of years younger by drilling close to an overhanging outcrop.[84]
The absorbed dose and dose equivalent from galactic cosmic rays and solar energetic particles on the Martian surface for ~300 days of observations during the current solar maximum was measured. These measurements are necessary for human missions to the surface of Mars, to provide microbial survival times of any possible extant or past life, and to determine how long potential organic biosignatures can be preserved. This study estimates that a 1-meter depth drill is necessary to access possible viable radioresistant microbe cells. The actual absorbed dose measured by the Radiation Assessment Detector (RAD) is 76 mGy/yr at the surface. Based on these measurements, for a round trip Mars surface mission with 180 days (each way) cruise, and 500 days on the Martian surface for this current solar cycle, an astronaut would be exposed to a total mission dose equivalent of ~1.01 sievert. Exposure to 1 sievert is associated with a 5 percent increase in risk for developing fatal cancer. NASA's current lifetime limit for increased risk for its astronauts operating in low-Earth orbit is 3 percent.[85] Maximum shielding from galactic cosmic rays can be obtained with about 3 meters of Martian soil.[86]
The samples examined were probably once mud that for millions to tens of millions of years could have hosted living organisms. This wet environment had neutral pH, low salinity, and variable redox states of both iron and sulfur species.[78][87][88][89] These types of iron and sulfur could have been used by living organisms.[90] C, H, O, S, N, and P were measured directly as key biogenic elements, and by inference, P is assumed to have been there as well.[81][83] The two samples, John Klein and Cumberland, contain basaltic minerals, Ca-sulfates, Fe oxide/hydroxides, Fe-sulfides, amorphous material, and trioctahedral smectites (a type of clay). Basaltic minerals in the mudstone are similar to those in nearby aeoliandeposits. However, the mudstone has far less Fe-forsterite plus magnetite, so Fe-forsterite (type of olivine) was probably altered to form smectite (a type of clay) and magnetite.[91] A Late Noachian/EarlyHesperian or younger age indicates that clay mineral formation on Mars extended beyond Noachian time; therefore, in this location neutral pH lasted longer than previously thought.[87]
In a press conference on December 8, 2014, Mars scientists discussed observations by Curiosity Rover that show Mars' Mount Sharp was built by sediments deposited in a large lake bed over tens of millions of years. This finding suggests the climate of ancient Mars could have produced long-lasting lakes at many places on the Planet. Rock layers indicate that a huge lake was filled and evaporated many times. The evidence was many deltas that were stacked upon each other.[92][93][94][95][96]
Also in December 2014, it was announced that Curiosity had detected sharp increases in methane four times out of twelve during a 20-month period with the Tunable Laser Spectrometer (TLS) of theSample Analysis at Mars instrument (SAM). Methane levels were ten times the usual amount. Due to the temporary nature of the methane spike, researchers believe the source is localized. The source may be biological or non-biological.[97][98][99]
On December 16, 2014, a team of researchers described how they have concluded that organic compounds have been found on Mars by Curiosity. The compounds were found in samples from drilling into Sheepbed mudstone. Chlorobenzene and several dichloroalkanes, such as dichloroethane, dichloropropane and dichlorobutane were discovered in the samples.[100][101]
On March 24, 2015, a paper was released describing the discovery of nitrates in three samples analysed by Curiosity. The nitrates are believed to have been created from diatomic nitrogen in the atmosphere during meteorite impacts.[102][103] Nitrogen is needed for all forms of life because it is used in the building blocks of larger molecules like DNA and RNA. Nitrates contain nitrogen in a form that can be used by living organisms; nitrogen in the air can not be used by organisms. This discovery of nitrates adds to the evidence that Mars once had life.[104][105]
The Jet Propulsion Laboratory announced in April 2015 the discovery of a network of two-tone mineral veins at an area called "Garden City" on lower Mount Sharp. The veins stand about 2.5 inches above the surface and are composed of two different minerals formed from at least two different fluid flows.[106] In Pahrump Hills, an area about 39 feet lower, the minerals clay, hematite, jarosite, quartz, and cristobalite were found.[107][108]
Measurements made by Curiosity allowed researchers to determine that Mars has liquid water at times. Because the humidity goes to 100% at night, salts, like calcium perchlorate, will absorb water from the air and form a brine in the soil. This process in which a salt absorbs water form the air is called deliquescence. Liquid water results even though the temperature is very low, as salts lower the freezing point of water. This principle is used when salt is spread on roads to melt snow/ice. The liquid brine produced in the night evaporates after sunrise. Much more liquid water is expected in higher latitudes where the colder temperature and more water vapor can result in higher levels of humidity more often.[109][110] The researchers cautioned that the amount of water was not enough to support life, but it could allow salts to move around in the soil.[111] The brimes would occur mostly in the upper 5 cm of the surface; however, there is evidence that the effects of liquid water can be detected down to 15 cm. Chlorine-bearing brines are corrosive; therefore design changes may need to be made for future landers.[112]
French and U.S. scientists found a type of granite by studying images and chemical results of 22 rock fragments. The composition of the rocks was determined with the ChemCam instrument. These pale rocks are rich in feldspar and may contain some quartz. The rocks are similar to Earth's granitic continental crust. They are like rocks called TTG (Tonalite-Trondhjemite-Granodiorite). On the Earth, TTG was common in the terrestrial continental crust in the Archean era (more than 2.5 billion years ago). By landing in Gale crater, Curiosity was able to sample a variety of rocks because the crater dug deep into the crust, thus exposing old rocks, some of which may be about 3.6 billion years old. For many years, Mars was thought to be composed of the dark, igneous rock basalt, so this is a significant discovery.[113][114][115]
On October 8, 2015, a large team of scientists confirmed the existence of long-lasting lakes in Gale Crater. The conclusion of Gale having lakes was based on evidence of old streams with coarser gravel in addition to places where streams appear to have emptied out into bodies of standing water. If lakes were once present, Curiosity would start seeing water-deposited, fine-grained rocks closer to Mount Sharp. That is exactly what happened.
Finely laminated mudstones were discovered by Curiosity; this lamination represents the settling of plumes of fine sediment through a standing body of water. Sediment deposited in a lake formed the lower portion of Mount Sharp, the mountain in Gale crater.[116][117][118]
At a press conference in San Francisco at the American Geophysical Union meeting, a group of scientists told of a discovery of very high concentrations of silica at some sites, along with the first ever discovery of a silica mineral called tridymite. The scientific team believes that water was involved with putting the silica in place. Acidic water would tend transport other ingredients away and leave silica behind, whereas alkaline or neutral water could carry in dissolved silica that would be deposited. This finding used measurements from ChemCam, the Alpha Particle X-ray Spectrometeter (APXS), and the Chemistry and Mineralogy (CheMin) instrument inside the rover. Tridymite was found in a rock named "Buckskin."[119] ChemCam and APXS measurements displayed high silica in pale zones along fractures in the bedrock beyond Marias Pass; hence silica may have been deposited by fluids that flowed through the fractures. CheMin found high silica levels in drilled material from a target called "Big Sky" and in another rock called "Greenhorn."[120]
As of the beginning of 2016, Curiosity had discovered seven hydrous minerals. The minerals are actinolite, montmorillonite, saponite, jarosite, halloysite, szomolnokite and magnesite. In some places the total concentration of all hydrous minerals was 40 vol%. Hydrous minerals help us understand the early water environment and possible biology on Mars. [121]
By using Curiosity's laser-firing device (ChemCam), scientists found manganese oxides in mineral veins in the "Kimberley" region of Gale Crater. These minerals need lots of water and oxidizing conditions to form; hence this discovery points to a water-rich, oxygen-rich past.[122] [123] [124]
A study of the kinds of minerals in veins examined with Curiosity found that evaporating lakes were present in the past in Gale crater. The Sheepbed Member mudstones of Yellowknife Bay (YKB) were examined in this research. [125] [126] [127]
Frost probably has formed in three locations in the first 1000 sols of the mission of the Curiosity exploration according to research published in Icarus in 2016.[128] This frost can cause weathering. Frost formation can explain the widespread detection of hydrated materials from orbit with the OMEGA instrument; it also can explain the hydrated component measured by Curiosity in Martian soil.[129] [130] [131]
Inverted Relief
Some places on Mars show inverted relief. In these locations, a stream bed may be a raised feature, instead of a valley. The inverted former stream channels may be caused by the deposition of large rocks or due to cementation. In either case erosion would erode the surrounding land but leave the old channel as a raised ridge because the ridge will be more resistant to erosion. An image below, taken with HiRISE shows sinuous ridges that may be old channels that have become inverted.[132]
-
Meandering Ridges that are probably inverted stream channels. Image taken with HiRISE.
-
CTX image of craters with black box showing location of next image.
-
Image from previous photo of a curved ridge that may be an old stream that has become inverted. Image taken with HiRISE under the HiWish program.
-
Sinuous Ridges within a branching fan in lower member of Medusae Fossae Formation, as seen by HiRISE.
Yardangs
Yardangs are common on Mars.[133] They are generally visible as a series of parallel linear ridges. Their parallel nature is thought to be caused by the direction of the prevailing wind. Two HiRISE images below show a good view of yardangs in the Aeolis quadrangle.[132] Yardangs are common in the Medusae Fossae Formation on Mars.
-
Stream channels in inverted relief and yardangs, as seen by HiRISE.
-
Aeolis Mensae Yardangs, as seen by HiRISE. Scale bar is 500 meters long. Click on image for better view of yardangs.
-
Medusae Fossae Formation southeast of Apollinaris Patera, as seen by HiRISE.
-
Yardangs in Medusae Fossae Formation with caprock labeled, as seen by HiRISE.
Layered terrain
Researchers, writing in Icarus, have described layered units in the Aeolis quadrangle at Aeolis Dorsa. A deposit that contains yardang was formed after several other deposits. The yardangs contain a layered deposit called "rhythmite" which was thought to be formed with regular changes in the climate. Because the layers appear harden, a damp or wet environment probably existed at the time. The authors correlate these layered deposits to the upper layers of Gale crater’s mound (Mt. Sharp).[134]
Many places on Mars show rocks arranged in layers. Sometimes the layers are of different colors. Light-toned rocks on Mars have been associated with hydrated minerals like sulfates. The Mars Rover Opportunity examined such layers close-up with several instruments. Some layers are probably made up of fine particles because they seem to break up into find dust. Other layers break up into large boulders so they are probably much harder. Basalt, a volcanic rock, is thought to in the layers that form boulders. Basalt has been identified on Mars in many places. Instruments on orbiting spacecraft have detected clay (also called phyllosilicate) in some layers. Recent research with an orbiting near-infrared spectrometer, which reveals the types of minerals present based on the wavelengths of light they absorb, found evidence of layers of both clay and sulfates in Columbus crater.[135] This is exactly what would appear if a large lake had slowly evaporated.[136] Moreover, because some layers contained gypsum, a sulfate which forms in relatively fresh water, life could have formed in the crater.[137]
Scientists are excited about finding hydrated minerals such as sulfates and clays on Mars because they are usually formed in the presence of water.[138] Places that contain clays and/or other hydrated minerals would be good places to look for evidence of life.[139]
Rock can form layers in a variety of ways. Volcanoes, wind, or water can produce layers.[140] Layers can be hardened by the action of groundwater. Martian ground water probably moved hundreds of kilometers, and in the process it dissolved many minerals from the rock it passed through. When ground water surfaces in low areas containing sediments, water evaporates in the thin atmosphere and leaves behind minerals as deposits and/or cementing agents. Consequently, layers of dust could not later easily erode away since they were cemented together. On Earth, mineral-rich waters often evaporate forming large deposits of various types of salts and other minerals. Sometimes water flows through Earth's aquifers, and then evaporates at the surface just as is hypothesed for Mars. One location this occurs on Earth is the Great Artesian Basin of Australia.[141] On Earth the hardness of many sedimentary rocks, like sandstone, is largely due to the cement that was put in place as water passed through.
-
Layers in lower member of Medusae Fossae Formation, as seen by HiRISE.
-
Buttes and layers in Aeolis, as seen by Mars Global Surveyor.
-
Layers along crater rim in Terra Sirenum, as seen by HiRIS under the HiWish program.
-
Layered terrain, as seen by HiRISE under HiWish program. Location is East of Gale Crater in the Aeolis quadrangle.
-
Layers and mounds in Medusae Fossae Formation, as seen by HiRISE under HiWish program Location is East of Gale Crater in the Aeolis quadrangle.
-
Layers and a field of small mounds Medusae Fossae Formation, as seen by HiRISE under HiWish program Location is East of Gale Crater in the Aeolis quadrangle.
-
Mound showing layers at the base, as seen by HiRISE under HiWish program Location is East of Gale Crater in the Aeolis quadrangle.
-
Layered structure, as seen by HiRISE under HiWish program
-
Layered features northeast of Gale Crater, as seen by HiRISE under HiWish program Layers may be similar to the many layers that are being examined by the Curiosity rover.
-
Wide view of layered terrain, as seen by HiRISE under HiWish program Location is northeast of Gale Crater.
-
Close view of mound with layers, as seen by HiRISE under HiWish program Note: this is an enlargement from the previous image.
-
Close view of mound with layers, as seen by HiRISE under HiWish program Note: this is an enlargement from a previous image.
-
Wide view of layered terrain, as seen by HiRISE under HiWish program Note: parts of this image are enlarged in the next three images.
-
Close view of layers in a mound, from previous image, as seen by HiRISE under HiWish program
-
Close view of layers in a mound, from a previous image, as seen by HiRISE under HiWish program
-
Close view of layers in a mound, from a previous image, as seen by HiRISE under HiWish program
Other features in Aeolis quadrangle
-
Possible fan or delta, as seen by HiRISE under HiWish program
-
Channel, as seen by HiRISE under HiWish program
-
Channels (indicated with arrows), as seen by HiRISE under HiWish program
Other Mars quadrangles
Interactive Mars map
See also
- Adirondack (Mars)
- Carbonates on Mars
- Climate of Mars
- Columbia Hills (Mars)
- Composition of Mars
- Curiosity (rover)
- Equatorial Layered Deposits
- Geology of Mars
- Groundwater on Mars
- Impact crater
- Inverted relief
- Jake Matijevic (rock)
- Lakes on Mars
- List of quadrangles on Mars
- List of rocks on Mars
- Martian soil
- Peace Vallis
- Scientific information from the Mars Exploration Rover mission
- Timeline of Mars Science Laboratory
- Water on Mars
- Yardangs on Mars
References
- ↑ Davies, M.E.; Batson, R.M.; Wu, S.S.C. "Geodesy and Cartography" in Kieffer, H.H.; Jakosky, B.M.; Snyder, C.W.; Matthews, M.S., Eds. Mars. University of Arizona Press: Tucson, 1992.
- ↑ NASA Staff (6 August 2012). "NASA Lands Car-Size Rover Beside Martian Mountain". NASA/JPL. Retrieved 2012-08-07.
- 1 2 Spirit rover follows up on scientific surprises
- 1 2 U.S. department of the Interior U.S. Geological Survey, Topographic Map of the Eastern Region of Mars M 15M 0/270 2AT, 1991
- 1 2 3 NASA Staff (27 March 2012). "'Mount Sharp' on Mars Compared to Three Big Mountains on Earth". NASA. Retrieved 31 March 2012.
- 1 2 3 Agle, D. C. (28 March 2012). "'Mount Sharp' On Mars Links Geology's Past and Future". NASA. Retrieved 31 March 2012.
- 1 2 3 Staff (29 March 2012). "NASA's New Mars Rover Will Explore Towering 'Mount Sharp'". Space.com. Retrieved 30 March 2012.
- 1 2 3 USGS (16 May 2012). "Three New Names Approved for Features on Mars". USGS. Retrieved 29 May 2012.
- ↑ Ori, G., I. Di Pietro, F. Salese. 2015. A WATERLOGGED MARTIAN ENVIRONMENT: CHANNEL PATTERNS AND SEDIMENTARY ENVIRONMENTS OF THE ZEPHYRIA ALLUVIAL PLAIN. 46th Lunar and Planetary Science Conference (2015) 2527.pdf
- ↑ McSween, etal. 2004. Basaltic Rocks Analyzed by the Spirit Rover in Gusev Crater. Science : 305. 842-845
- 1 2 Arvidson, R. E., et al. (2004) Science, 305, 821-824
- ↑ Gelbert, R., et al. 2006. The Alpha Particle X-ray Spectrometer (APXS): results from Gusev crater and calibration report. J. Geophys. Res. – Planets: 111.
- ↑ Christensen, P. Initial Results from the Mini-TES Experiment in Gusev Crater from the Spirit Rover. Science: 305. 837-842.
- ↑ Bertelsen, P., et al. 2004. Magnetic Properties on the Mars Exploration Rover Spirit at Gusev Crater. Science: 305. 827-829
- 1 2 Bell, J (ed.) The Martian Surface. 2008. Cambridge University Press. ISBN 978-0-521-86698-9
- ↑ Gelbert, R. et al. Chemistry of Rocks and Soils in Gusev Crater from the Alpha Particle X-ray Spectrometer. Science: 305. 829-305
- 1 2 McSween, etal. 2004. Basaltic Rocks Analyzed by the Spirit Rover in Gusev Crater. Science : 305. 842-845
- ↑ Squyres, S., et al. 2006 Rocks of the Columbia Hills. J. Geophys. Res – Planets. 111
- ↑ Ming,D., et al. 2006 Geochemical and mineralogical indicators for aqueous processes in the Columbia Hills of Gusev crater, Mars. J. Geophys: Res.111
- 1 2 Schroder, C., et al. (2005) European Geosciences Union, General Assembly, Geophysical Research abstr., Vol. 7, 10254, 2005
- ↑ Christensen, P.R. (2005) Mineral Composition and Abundance of the Rocks and Soils at Gusev and Meridiani from the Mars Exploration Rover Mini-TES Instruments AGU Joint Assembly, 23–27 May 2005 http://www.agu.org/meetings/sm05/waissm05.html
- ↑ http://spaceref.com/mars/signs-of-acid-fog-found-on-mars.html
- ↑ https://gsa.confex.com/gsa/2015AM/webprogram/Paper266774.html
- ↑ COLE, Shoshanna B., et al. 2015. IN-SITU EVIDENCE FOR ALTERATION BY ACID FOG ON HUSBAND HILL, GUSEV CRATER, MARS. 2015 GSA Annual Meeting in Baltimore, Maryland, USA (1–4 November 2015) Paper No. 94-10
- ↑ Klingelhofer, G., et al. (2005) Lunar Planet. Sci. XXXVI abstr. 2349
- ↑ Morris,S., et al. Mossbauer mineralogy of rock, soil, and dust at Gusev crater, Mars: Spirit’s journal through weakly altered olivine basalt on the plains and pervasively altered basalt in the Columbia Hills. J. Geophys. Res: 111
- ↑ Ming,D., et al. 2006 Geochemical and mineralogical indicators for aqueous processes in the Columbia Hills of Gusev crater, Mars. J. Geophys. Res.111
- ↑ http://www.nasa.gov/mission_pages/mer/mer-20070521.html
- ↑ http://www.sciencedaily.com/releases/2010/06/100603140959.htm
- ↑ Richard V. Morris, Steven W. Ruff, Ralf Gellert, Douglas W. Ming, Raymond E. Arvidson, Benton C. Clark, D. C. Golden, Kirsten Siebach, Göstar Klingelhöfer, Christian Schröder, Iris Fleischer, Albert S. Yen, Steven W. Squyres. Identification of Carbonate-Rich Outcrops on Mars by the Spirit Rover. Science, June 3, 2010 doi:10.1126/science.1189667 PMID 20522738
- ↑ Cabrol, N. and E. Grin (eds.). 2010. Lakes on Mars. Elsevier. NY.
- ↑ Rossman, R.; et al. (2002). "A large paleolake basin at the head of Ma'adim Vallis, Mars". Science. 296: 2209–2212. Bibcode:2002Sci...296.2209I. doi:10.1126/science.1071143. PMID 12077414.
- ↑ http://www.uahirise.org/ESP_037142_1430
- ↑ Rossman, P. Irwin III; Ted A. Maxwell; Alan D. Howard; Robert A. Craddock; David W. Leverington (21 June 2002). "A Large Paleolake Basin at the Head of Ma'adim Vallis, Mars". Science. 296 (5576): 2209–2212. Bibcode:2002Sci...296.2209I. doi:10.1126/science.1071143. PMID 12077414.
- ↑ http://antwrp.gsfc.nasa.gov/apod/ap020627.html
- ↑ Baker, V. 1982. The Channels of Mars. Univ. of Tex. Press, Austin, TX
- ↑ Baker, V.; Strom, R.; Gulick, V.; Kargel, J.; Komatsu, G.; Kale, V. (1991). "Ancient oceans, ice sheets and the hydrological cycle on Mars". Nature. 352: 589–594. Bibcode:1991Natur.352..589B. doi:10.1038/352589a0.
- ↑ Carr, M (1979). "Formation of Martian flood features by release of water from confined aquifers". J. Geophys. Res. 84: 2995–300. Bibcode:1979JGR....84.2995C. doi:10.1029/jb084ib06p02995.
- ↑ Komar, P (1979). "Comparisons of the hydraulics of water flows in Martian outflow channels with flows of similar scale on Earth". Icarus. 37: 156–181. Bibcode:1979Icar...37..156K. doi:10.1016/0019-1035(79)90123-4.
- 1 2 Hugh H. Kieffer (1992). Mars. University of Arizona Press. ISBN 978-0-8165-1257-7. Retrieved 7 March 2011.
- ↑ Raeburn, P. 1998. Uncovering the Secrets of the Red Planet Mars. National Geographic Society. Washington D.C.
- ↑ Moore, P. et al. 1990. The Atlas of the Solar System. Mitchell Beazley Publishers NY, NY.
- ↑ Carr, M (1979). "Formation of martian flood features by release of water from confined aquifers". J. Geophys. Res. 84: 2995–3007. Bibcode:1979JGR....84.2995C. doi:10.1029/jb084ib06p02995.
- ↑ Hanna, J. and R. Phillips. 2005. Tectonic pressurization of aquifers in the formation of Mangala and Athabasca Valles on Mars. LPSC XXXVI. Abstract 2261.
- ↑ http://hirise.lpl.arizona.edu/PSP_008437_1750
- ↑ http://mars.jpl.nasa.gov/mgs/msss/camera/images/dec00_seds/slides/265L/
- ↑ Milliken, R. et al. 2010. Paleoclimate of Mars as captured by the stratigraphic record in Gale Crater. Geophysical Research Letters. 37: L04201
- ↑ Thompson, B.; et al. (2011). "Constraints on the origin and evolution of the layered mound in Gale Crater, Mars using Mars Reconnaissance Orbiter data". Icarus. 214: 413–432. Bibcode:2011Icar..214..413T. doi:10.1016/j.icarus.2011.05.002.
- ↑ Grotzinger, J. and R. Milliken. 2012. Sedimentary Geology of Mars. SEPM.
- ↑ http://www.msss.com/mars_images/moc/dec00_seds/slides/265E
- ↑ IAU Staff (September 26, 2012). "Gazeteer of Planetary Nomenclature: Peace Vallis". IAU. Retrieved September 28, 2012.
- 1 2 Brown, Dwayne; Cole, Steve; Webster, Guy; Agle, D.C. (September 27, 2012). "NASA Rover Finds Old Streambed On Martian Surface". NASA. Retrieved September 28, 2012.
- 1 2 NASA (September 27, 2012). "NASA's Curiosity Rover Finds Old Streambed on Mars - video (51:40)". NASAtelevision. Retrieved September 28, 2012.
- 1 2 Chang, Alicia (September 27, 2012). "Mars rover Curiosity finds signs of ancient stream". AP News. Retrieved September 27, 2012.
- ↑ Chang, Kenneth (December 9, 2013). "On Mars, an Ancient Lake and Perhaps Life". New York Times. Retrieved December 9, 2013.
- ↑ Various (December 9, 2013). "Science - Special Collection - Curiosity Rover on Mars". Science. Retrieved December 9, 2013.
- ↑ Dietrich, W., M. Palucis, T. Parker, D. Rubin, K.Lewis, D. Sumner, R. Williams. 2014. Clues to the relative timing of lakes in Gale Crater. Eighth International Conference on Mars (2014) 1178.pdf.
- ↑ http://www.lpi.usra.edu/publications/slidesets/stones/
- ↑ http://themis.asu.edu/features/ianichaos
- ↑ http://dsc.discovery.com/news/2008/11/21/mars-landing-sites-02.html
- ↑ http://www.space.com/missionlaunches/mars-science-laboratory-curiosity-landing-sites-100615.htm
- ↑ http://pubs.er.usgs.gov/publication/70047207
- ↑ Williams, R., et al. 2013. Martian fluvial conglomerates at Gale Crater. doi:10.1126/science.1237317
- ↑ Brown, Dwayne (October 30, 2012). "NASA Rover's First Soil Studies Help Fingerprint Martian Minerals". NASA. Retrieved October 31, 2012.
- 1 2 Brown, Dwayne; Webster, Guy; Neal-Jones, Nancy (December 3, 2012). "NASA Mars Rover Fully Analyzes First Martian Soil Samples". NASA. Retrieved December 3, 2012.
- 1 2 Chang, Ken (December 3, 2012). "Mars Rover Discovery Revealed". New York Times. Retrieved December 3, 2012.
- 1 2 Webster, Guy; Brown, Dwayne (March 18, 2013). "Curiosity Mars Rover Sees Trend In Water Presence". NASA. Retrieved March 20, 2013.
- ↑ Rincon, Paul (March 19, 2013). "Curiosity breaks rock to reveal dazzling white interior". BBC. Retrieved March 19, 2013.
- ↑ Staff (March 20, 2013). "Red planet coughs up a white rock, and scientists freak out". MSN. Retrieved March 20, 2013.
- 1 2 Agle, DC; Brown, Dwayne (March 12, 2013). "NASA Rover Finds Conditions Once Suited for Ancient Life on Mars". NASA. Retrieved March 12, 2013.
- 1 2 Wall, Mike (March 12, 2013). "Mars Could Once Have Supported Life: What You Need to Know". Space.com. Retrieved March 12, 2013.
- 1 2 Chang, Kenneth (March 12, 2013). "Mars Could Once Have Supported Life, NASA Says". New York Times. Retrieved March 12, 2013.
- ↑ Harwood, William (March 12, 2013). "Mars rover finds habitable environment in distant past". Spaceflightnow. Retrieved March 12, 2013.
- ↑ Grenoble, Ryan (March 12, 2013). "Life On Mars Evidence? NASA's Curiosity Rover Finds Essential Ingredients In Ancient Rock Sample". Huffington Post. Retrieved March 12, 2013.
- ↑ Stolper, E.; et al. (2013). "The Petrochemistry of Jake M: A Martian Mugearite.". Science. 341: 6153. doi:10.1126/science.1239463.
- ↑ Blake, D.; et al. (2013). "Curiosity at Gale crater, Mars: characterization and analysis of the Rocknest sand shadow - Medline". Science. 341: 1239505. doi:10.1126/science.1239505.
- ↑ Leshin, L.; et al. (2013). "Volatile, isotope, and organic analysis of martian fines with the Mars Curiosity rover - Medline". Science. 341: 1238937. doi:10.1126/science.1238937.
- 1 2 McLennan, M.; et al. (2013). "Elemental geochemistry of sedimentary rocks at Yellowknife Bay, Gale Crater, Mars.". Science. 343: 1244734. Bibcode:2014Sci...343C.386M. doi:10.1126/science.1244734.
- ↑ Flynn, G. (1996). "The delivery of organic matter from asteroids and comets to the early surface of Mars.". Earth Moon Planets - Medline. 72: 469–474. Bibcode:1996EM&P...72..469F. doi:10.1007/BF00117551.
- ↑ Benner, S.; K.Devine; L. Matveeva; D. Powell. (2000). "The missing organic molecules on Mars - Medline". Proc. Natl. Acad. Sci. U.S.A. 97: 2425–2430. Bibcode:2000PNAS...97.2425B. doi:10.1073/pnas.040539497. PMC 15945
. PMID 10706606.
- 1 2 Grotzinger, J.; et al. (2013). "A Habitable Fluvio-Lacustrine Environment at Yellowknife Bay, Gale Crater, Mars". Science. 343: 1242777. Bibcode:2014Sci...343A.386G. doi:10.1126/science.1242777. PMID 24324272.
- ↑ Kerr, R.; et al. (2013). "New Results Send Mars Rover on a Quest for Ancient Life.". Science. 342 (6164): 1300–1301. doi:10.1126/science.342.6164.1300. PMID 24337267.
- 1 2 Ming, D.; et al. (2013). "Volatile and Organic Compositions of Sedimentary Rocks in Yellowknife Bay, Gale Crater, Mars.". Science. 343: 1245267. Bibcode:2014Sci...343E.386M. doi:10.1126/science.1245267.
- ↑ Farley, K.; et al. (2013). "In Situ Radiometric and Exposure Age Dating of the Martian Surface.". Science. 343: 1247166. Bibcode:2014Sci...343F.386H. doi:10.1126/science.1247166.
- ↑ Staff (December 9, 2013). "Understanding Mars' Past and Current Environments". NASA. Retrieved December 20, 2013.
- ↑ Hassler, D.; et al. (2013). "Mars' Surface Radiation Environment Measured with the Mars Science Laboratory's Curiosity Rover.". Science. 343 (6169): 1244797. Bibcode:2014Sci...343D.386H. doi:10.1126/science.1244797. PMID 24324275.
- 1 2 Vaniman, D.; et al. (2013). "Mineralogy of a mudstone at Yellowknife Bay, Gale crater, Mars.". Science. 343: 1243480. Bibcode:2014Sci...343B.386V. doi:10.1126/science.1243480.
- ↑ Bibring, J.; et al. (2006). "Global mineralogical and aqueous mars history derived from OMEGA/Mars Express data. Medline". Science. 312 (5772): 400–404. Bibcode:2006Sci...312..400B. doi:10.1126/science.1122659. PMID 16627738.
- ↑ Squyres, S.; A. Knoll. (2005). "Sedimentary rocks and Meridiani Planum: Origin, diagenesis, and implications for life of Mars. Earth Planet.". Sci. Lett. 240: 1–10. Bibcode:2005E&PSL.240....1S. doi:10.1016/j.epsl.2005.09.038.
- ↑ Nealson, K.; P. Conrad. (1999). "Life: past, present and future. Medline.". Phil. Trans. R. Soc. Lond. B. 354: 1923–1939.
- ↑ Keller, L.; et al. (1994). "Aqueous alteration of the Bali CV3 chondrite: Evidence from mineralogy, mineral chemistry, and oxygen isotopic compositions. Medline.". Geochim. Cosmochim. Acta. 58 (24): 5589–5598. Bibcode:1994GeCoA..58.5589K. doi:10.1016/0016-7037(94)90252-6. PMID 11539152.
- ↑ Brown, Dwayne; Webster, Guy (December 8, 2014). "Release 14-326 - NASA's Curiosity Rover Finds Clues to How Water Helped Shape Martian Landscape". NASA. Retrieved December 8, 2014.
- ↑ Kaufmann, Marc (December 8, 2014). "(Stronger) Signs of Life on Mars". New York Times. Retrieved December 8, 2014.
- ↑ http://www.sciencedaily.com/releases/2014/12/141208122903.htm
- ↑ http://www.jpl.nasa.gov/video/details.php?id=1346
- ↑ http://www.jpl.nasa.gov/news/news.php?feature=4398
- ↑ http://www.nasa.gov/press/2014/december/nasa-rover-finds-active-ancient-organic-chemistry-on-mars/#.VJL_2F4AAC
- ↑ Webster1, C. et al. 2014. Mars methane detection and variability at Gale crate. Science. 1261713
- ↑ http://www.nbclosangeles.com/news/local/Mars-Rover-Curiosity-Methane-285996081.html?utm_source=Explore+Mars+Newsletter&utm_campaign=46650445b1-December12_4_2014&utm_medium=email&utm_term=0_c4f9e110c3-46650445b1-30671213
- ↑ http://www.sciencedaily.com/releases/2014/12/141216144137.htm
- ↑ http://www.nasa.gov/content/goddard/mars-organic-matter/#.VJB9o3vX1a8
- ↑ http://www.sciencedaily.com/releases/2015/03/150325082341.htm
- ↑ Stern, J., B. Sutter, C. Freissinet, R. Navarro-González, C. McKay, P. Archer, A. Buch, A. Brunner, P. Coll, J. Eigenbrode, A. Fairen, H. Franz, D. Glavin, Srishti Kashyap, A. McAdam, D. Ming, A. Steele, C. Szopa, J. Wray, F. Martín-Torres, Maria-Paz Zorzano, P. Conrad, P. Mahaffy. Evidence for indigenous nitrogen in sedimentary and aeolian deposits from the Curiosityrover investigations at Gale crater, Mars" Proceedings of the National Academy of Sciences 2015; 201420932 doi:10.1073/pnas.1420932112
- ↑ http://astrobiology.com/2015/03/curiosity-rover-finds-biologically-useful-nitrogen-on-mars.html
- ↑ http://www.space.com/28899-mars-life-nitrogen-carbon-monoxide.html?cmpid=514630_20150324_42568376&adbid=10152715032851466&adbpl=fb&adbpr=17610706465
- ↑ http://www.space.com/29001-mars-rover-curiosity-ice-cream-rocks.html?cmpid=NL_SP_weekly_2015-04-03
- ↑ http://www.jpl.nasa.gov/news/news.php?feature=4536&utm_source=iContact&utm_medium=email&utm_campaign=NASAJPL&utm_content=daily20150401-3
- ↑ http://www.nasa.gov/mission_pages/msl/index.html
- ↑ http://www.jpl.nasa.gov/news/news.php?feature=4549&utm_source=iContact&utm_medium=email&utm_campaign=NASAJPL&utm_content=daily20150413-3
- ↑ University of Copenhagen - Niels Bohr Institute. "Mars might have salty liquid water." ScienceDaily. ScienceDaily, 13 April 2015. <www.sciencedaily.com/releases/2015/04/150413130611.htm>.
- ↑ http://www.space.com/29072-mars-liquid-water-at-night.html?cmpid=NL_SP_weekly_2015-04-13
- ↑ Martin-Torre, F. et al. 2015. Transient liquid water and water activity at Gale crater on Mars. Nature geoscienceDOI:10.1038/NGEO2412
- ↑ http://spaceref.com/mars/evidence-of-mars-primitive-continental-crust.html
- ↑ http://www.sciencedaily.com/releases/2015/07/150714142051.htm
- ↑ Sautter, V., M. Toplis, R. Wiens, A. Cousin, C. Fabre, O. Gasnault, S. Maurice, O. Forni, J. Lasue, A. Ollila, J. Bridges, N. Mangold, S. Le Mouélic, M. Fisk, P.-Y. Meslin, P. Beck, P. Pinet, L. Le Deit, W. Rapin, E. Stolper, H. Newsom, D. Dyar, N. Lanza, D. Vaniman, S. Clegg, J. Wray. In situ evidence for continental crust on early Mars. Nature Geoscience, 2015; DOI: 10.1038/ngeo2474
- ↑ http://astrobiology.com/2015/10/wet-paleoclimate-of-mars-revealed-by-ancient-lakes-at-gale-crater.html>
- ↑ Clavin, Whitney (October 8, 2015). "NASA's Curiosity Rover Team Confirms Ancient Lakes on Mars". NASA. Retrieved October 9, 2015.
- ↑ Grotzinger, J.P.; et al. (October 9, 2015). "Deposition, exhumation, and paleoclimate of an ancient lake deposit, Gale crater, Mars". Science. 350 (6257). Bibcode:2015Sci...350.7575G. doi:10.1126/science.aac7575. Retrieved October 9, 2015.
- ↑ DOE/Los Alamos National Laboratory. "New Mars rover findings revealed: Much higher concentrations of silica indicate 'considerable water activity'." ScienceDaily. ScienceDaily, 17 December 2015. <www.sciencedaily.com/releases/2015/12/151217143352.htm>.
- ↑ http://spaceref.com/mars/high-concentrations-of-silica-indicate-considerable-water-activity-on-mars.html
- ↑ Lin, H. et al. 2016. Abundance retrieval of hydrous minerals around the Mars Science Laboratory landing site in Gale crater, Mars. Planetary and Space Science: 121, 76-82.
- ↑ NASA/Jet Propulsion Laboratory. "NASA rover findings point to a more Earth-like Martian past." ScienceDaily. ScienceDaily, 27 June 2016. <www.sciencedaily.com/releases/2016/06/160627125731.htm>.
- ↑ 1.Nina L. Lanza, Roger C. Wiens, Raymond E. Arvidson, Benton C. Clark, Woodward W. Fischer, Ralf Gellert, John P. Grotzinger, Joel A. Hurowitz, Scott M. McLennan, Richard V. Morris, Melissa S. Rice, James F. Bell, Jeffrey A. Berger, Diana L. Blaney, Nathan T. Bridges, Fred Calef, John L. Campbell, Samuel M. Clegg, Agnes Cousin, Kenneth S. Edgett, Cécile Fabre, Martin R. Fisk, Olivier Forni, Jens Frydenvang, Keian R. Hardy, Craig Hardgrove, Jeffrey R. Johnson, Jeremie Lasue, Stéphane Le Mouélic, Michael C. Malin, Nicolas Mangold, Javier Martìn-Torres, Sylvestre Maurice, Marie J. McBride, Douglas W. Ming, Horton E. Newsom, Ann M. Ollila, Violaine Sautter, Susanne Schröder, Lucy M. Thompson, Allan H. Treiman, Scott VanBommel, David T. Vaniman, Marìa-Paz Zorzano. Oxidation of manganese in an ancient aquifer, Kimberley formation, Gale crater, Mars. Geophysical Research Letters, 2016; DOI: 10.1002/2016GL069109
- ↑ http://www.jpl.nasa.gov/news/news.php?feature=6544&utm_source=iContact&utm_medium=email&utm_campaign=NASAJPL&utm_content=daily20160627
- ↑ http://onlinelibrary.wiley.com/doi/10.1111/maps.12668/abstract;jsessionid=C356059B3DF8928987AC75CBEB53FC03.f04t01
- ↑ Schwenzer, S. et al. 2016. Fluids during diagenesis and sulfate vein formation in sediments at Gale crater, Mars. Meteoritics & Planetary Science. DOI: 10.1111/maps.12668
- ↑ https://www.sciencedaily.com/releases/2016/08/160805085749.htm
- ↑ Martinex, G. et al. 2016. Likely frost events at Gale crater: Analysis from MSL/REMS measurements. Icarus: 280, 93-102.
- ↑ Audouard, J. et al. 2014. Water in the martian regolith from OMEGA/Mars Express. J. Geophys. Res: Planets. 8, 1969-1989.
- ↑ Leshin, L. 2013. Volatile, isotope, and organic analysis of martian fines with the Mars Curiosity rover. Science: 341, 6153.
- ↑ Meslin, P., et al. 2013. Soil diversity and hydration as observed by ChemCam at Gale crater, Mars. Science: 341, 6153.
- 1 2 http://hiroc.lpl.arizona.edu/images/PSP/diafotizo.php?ID=PSP_002279_1735
- ↑ Grotzinger, J. and R. Milliken (eds.) 2012. Sedimentary Geology of Mars. SEPM
- ↑ Kite, E., A. Howard, A. Lucas, J. Armstrong, O.Aharonson, M. Lamb. 2015. Stratigraphy of Aeolis Dorsa, Mars: Stratigraphic context of the great river deposits. Icarus: 253, 223–242
- ↑ Cabrol, N. and E. Grin (eds.). 2010. Lakes on Mars. Elsevier.NY.
- ↑ Wray, J. et al. 2009. Columbus Crater and other possible plaelakes in Terra Sirenum, Mars. Lunar and Planetary Science Conference. 40: 1896.
- ↑ "Martian "Lake Michigan" Filled Crater, Minerals Hint". News.nationalgeographic.com. 2010-10-28. Retrieved 2012-08-04.
- ↑ "Target Zone: Nilosyrtis? | Mars Odyssey Mission THEMIS". Themis.asu.edu. Retrieved 2012-08-04.
- ↑ "HiRISE | Craters and Valleys in the Elysium Fossae (PSP_004046_2080)". Hirise.lpl.arizona.edu. Retrieved 2012-08-04.
- ↑ "HiRISE | High Resolution Imaging Science Experiment". Hirise.lpl.arizona.edu?psp_008437_1750. Retrieved 2012-08-04.
- ↑ Habermehl, M. A. (1980) The Great Artesian Basin, Australia. J. Austr. Geol. Geophys. 5, 9–38.
- 1 2 Morton, Oliver (2002). Mapping Mars: Science, Imagination, and the Birth of a World. New York: Picador USA. p. 98. ISBN 0-312-24551-3.
- ↑ "Online Atlas of Mars". Ralphaeschliman.com. Retrieved December 16, 2012.
- ↑ "PIA03467: The MGS MOC Wide Angle Map of Mars". Photojournal. NASA / Jet Propulsion Laboratory. February 16, 2002. Retrieved December 16, 2012.
- ↑ "Online Atlas of Mars". Ralphaeschliman.com. Retrieved December 16, 2012.
- ↑ "PIA03467: The MGS MOC Wide Angle Map of Mars". Photojournal. NASA / Jet Propulsion Laboratory. February 16, 2002. Retrieved December 16, 2012.
Further reading
- Grotzinger, J. and R. Milliken (eds.). 2012. Sedimentary Geology of Mars. SEPM.
- Lakdawalla, E. 2011. Target: Gale Curiosity Will Soon Have a New Home. The Planetary Report. 31 (4), 15-21
External links
![]() |
Wikimedia Commons has media related to Aeolis quadrangle. |
- Video (04:32) - Evidence: Water "Vigorously" Flowed On Mars - September, 2012
- Lakes, Fans, Deltas and Streams: Geomorphic Constraints ...
- Lakes on Mars - Nathalie Cabrol (SETI Talks)
Quadrangles on Mars | ||||||||||||||||||||||||||||||||||||||||||||||||||||||||||||||||||||||||||||||||||||||||||||||||||||||||||||||||||||||||||||||||||||||||||||||||
---|---|---|---|---|---|---|---|---|---|---|---|---|---|---|---|---|---|---|---|---|---|---|---|---|---|---|---|---|---|---|---|---|---|---|---|---|---|---|---|---|---|---|---|---|---|---|---|---|---|---|---|---|---|---|---|---|---|---|---|---|---|---|---|---|---|---|---|---|---|---|---|---|---|---|---|---|---|---|---|---|---|---|---|---|---|---|---|---|---|---|---|---|---|---|---|---|---|---|---|---|---|---|---|---|---|---|---|---|---|---|---|---|---|---|---|---|---|---|---|---|---|---|---|---|---|---|---|---|---|---|---|---|---|---|---|---|---|---|---|---|---|---|---|---|
|