Lakes on Mars
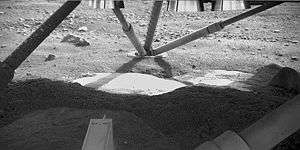
In summer 1965, the first close-up pictures from Mars showed a cratered desert with no signs of water.[1][2][3] However, over the decades, as more parts of the planet were imaged with better cameras on more sophisticated satellites, Mars showed evidence of past river valleys, lakes, and ice in glaciers and in the ground.[4] It was discovered that the climate of Mars displays huge changes over geologic time because its axis is not stabilized by a large moon, as Earth's is.[5][6][7] Also, some researchers maintain that water could exist for periods of time due to geothermal effects or asteroid impacts.[8][9][10][11][12]
Besides seeing features that were signs of past water, researchers found other types of evidence for past water. Minerals detected in many locations needed water to form.[13][14][15][16][17] An instrument in the Mars Odyssey, an orbiting spacecraft, mapped the distribution of water in the near surface.[18][19][20] When the Phoenix spacecraft fired its rockets to land in the far north, ice was exposed.[21][22]
When water enters a large body of water, such as a lake, a delta may form. Many craters and other depressions on Mars show deltas that resemble those on Earth. In addition, if a lake lies in a depression, channels entering it will all stop at the same altitude. Such an arrangement is visible around places on Mars that are supposed to have contained large bodies of water—including around a possible ocean in the North.
Lake formation has been proposed by various researchers for quite some time.[23][24][25] One study found 205 possible closed-basin lakes in craters on Mars. The basins have an inlet valley that cuts the crater rim and flows into the basin, but they have no visible outlet valley. The total volume of the basins is equivalent to a depth of 1.2 meters spread evenly over the Martian surface. However, this amount is a small fraction of the current water ice stores on Mars.[26] Another study found 210 open-basin lakes. These were lakes with both an inlet and an outlet; hence water must have entered the basin, and reached the height of the outlet. Some of these lakes had volumes similar to Earth's Caspian Sea, Black Sea, and Lake Baikal.[27]
Moreover, some basins on Mars form part of long chains of lakes.[24] The Naktong/Scamander/Mamers Valles lake-chain system is about 4500 km (2800 miles) long, with a drainage area similar to that of the Missouri-Mississippi rivers.[28] Another, the Samara/Himera Vallis system, is 1800 km long.[29] Many of the long chains of lakes are found in the Margaritifer Sinus quadrangle.[30]
Some of the lakes appear to have had a high volume as compared to their drainage area; hence, it is believed that some of the water was groundwater. Further evidence is the existence of knobby material on the basin floors. These knobs can be formed when large amounts of water left the ground.[31][32][33]
This article describes some of the places that could have held large lakes.
Images of possible deltas
- Possible delta in Margaritifer Sinus quadrangle as seen by THEMIS.
- Probable delta in Eberswalde Crater that lies to the NE of Holden Crater, as seen by Mars Global Surveyor. Image in Margaritifer Sinus quadrangle.
- Delta in Lunae Palus quadrangle, as seen by THEMIS.
- Delta that fills a crater in Lunae Palus quadrangle, as seen by HiRISE.
Mars Ocean


The Mars ocean hypothesis states that nearly a third of the surface of Mars was covered by an ocean of liquid water early in the planet’s geologic history.[35][36] This primordial ocean, dubbed Paleo-Ocean[34] and Oceanus Borealis,[37] would have filled the Vastitas Borealis basin in the northern hemisphere, a region which lies 4–5 km (2.5–3 miles) below the mean planetary elevation, about 3.8 billion years ago. Evidence for this ocean includes geographic features resembling ancient shorelines, and the chemical properties of the Martian soil and atmosphere.[38][39][40] However, for such an ocean to have existed, early Mars would have required a denser atmosphere and warmer climate to allow liquid water to remain at the surface.[41]
History of observational evidence for Martian oceans
Features shown by the Viking orbiters in 1976 revealed two possible ancient shorelines near the pole, Arabia and Deuteronilus, each thousands of kilometers long.[42] Several physical features in the present geography of Mars suggest the past existence of a primordial ocean. Networks of gullies that merge into larger channels imply erosion by a liquid flow, and resemble ancient riverbeds on Earth. Enormous channels, 25 km wide and several hundred meters deep, appear to have flowed directly from underground aquifers in the Southern uplands into the Northern plains.[41] Much of the northern hemisphere of Mars is at a significantly lower elevation than the rest of the planet (the Martian dichotomy), and is unusually flat. The low elevation would cause water, if it existed, to gather there. An ocean would tend to level out the ground underneath it.
The acceptance of a vast northern ocean has waxed and waned over the decades. Beginning in 1998, scientists Michael Malin and Kenneth Edgett set out to investigate, using cameras on board the Mars Global Surveyor with a resolution five to ten times better than those of the Viking spacecraft, in places that would test shorelines proposed by others in the scientific literature.[43] Their analysis was inconclusive at best, and reported that the shoreline varies in elevation by several kilometers, rising and falling from one peak to the next for thousands of miles.[44] This report cast doubt on whether the features truly mark a long-gone sea coast, and has been taken as an argument against the Martian shoreline (and ocean) hypothesis.
Research published in 2009 shows a much higher density of stream channels than formerly believed. Regions on Mars with the most valleys are comparable to what is found on Earth. The research team developed a computer program to identify valleys by searching in topographical data for U-shaped structures.[45] The large extent of valley networks found strongly supports rain on the planet in the past. The global pattern of the Martian valleys could be explained by a big northern ocean. A large ocean in the northern hemisphere would explain why there is a southern limit to valley networks: the southernmost regions of Mars, farthest from the water reservoir, would get little rainfall and would develop no valleys. Similarly, the lack of rainfall would explain why Martian valleys become shallower from north to south.[46] A 2010 study of deltas on Mars revealed that seventeen of them are found at the altitude of a proposed shoreline for a Martian ocean.[47] This is what would be expected if the deltas were all next to a large body of water.[48] Research published in 2012 using data from MARSIS, a radar on board the Mars Express orbiter, supports the hypothesis of a former large northern ocean. The instrument revealed a dielectric constant of the surface similar to those of low-density sedimentary deposits, massive deposits of ground-ice, or a combination of the two. The measurements were not like those of a lava-rich surface.[49]
In March 2015, scientists stated that evidence exists for an ancient volume of water that could comprise an ocean, likely in the planet's northern hemisphere and about the size of Earth's Arctic Ocean.[50] This finding was derived from the ratio of water and deuterium in the modern Martian atmosphere compared to the ratio found on Earth and derived from telescopic observations. Eight times as much deuterium was inferred at the polar deposits of Mars than exists on Earth (VSMOW), suggesting that ancient Mars had significantly higher levels of water. The representative atmospheric value obtained from the maps (7 VSMOW) is not affected by climatological effects as those measured by localized rovers, although the telescopic measurements are within range to the enrichment measured by the Curiosity rover in Gale Crater of 5-7 VSMOW.[51]
Valles Marineris canyon system
Map of Coprates quadrangle from Mars Orbiter Laser Altimeter (MOLA) data. The highest elevations are red and the lowest are blue. | |
Coordinates | 15°00′S 67°30′W / 15°S 67.5°WCoordinates: 15°00′S 67°30′W / 15°S 67.5°W |
---|
Valles Marineris is the largest canyon system in the solar system, and much evidence suggests that all or parts of the canyon system contained lakes. It is located in the Coprates quadrangle. The walls of the canyons often contain many layers. The floors of some of the canyons contain large deposits of layered materials. Some researchers believe that the layers were formed when water once filled the canyons.[35][52][53][54] Layered deposits, called interior layered deposits (ILDs), in various parts of Valles Marineris, especially Candor Chasma and Juventae Chasma, have led many researchers to believe that they were formed when the whole area was a giant lake. However, many other ideas have been advanced to explain them.[55] High-resolution structural and geologic mapping in west Candor Chasma, presented in March 2015, showed that the deposits on the floor of the Candor chasma are basin-filling sediments that were deposited in a wet playa-like setting; hence water was involved in their formation.[56] Minerals that generally require water for their formation have been found in ILDs, thus supporting water in the system. The European Space Agency's Mars Express found possible evidence for the sulfates epsomite and kieserite, minerals that form in water.[57] Ferric oxide in the form of crystalline grey hematite, which typically requires water for its formation, was also detected.[35][58][59] Although there is much controversy about a lake in the whole of Valles Marineris, a fairly strong case can be made for smaller lakes. Melas Chasma is especially believed to have once contained a lake, as it is deepest part of the Valles Marineris system at 11 km (7 miles) below the surrounding surface. From here to the outflow channels there is about a 0.03 degree slope upward to the northern plains, which means that if the canyon was filled with liquid, there would be a lake 1 km deep before the fluid would flow out onto the northern plains.[60] Melas Chasma is the widest segment of the Valles Marineris canyon system,[61] located east of Ius Chasma at 9.8°S, 283.6°E in Coprates quadrangle. It cuts through layered deposits that are thought to be sediments from an old lake that resulted from runoff of the valley networks to the west.[62] Support for abundant past water in Melas Chasma comes from the discovery by MRO of hydrated sulfates that need water for their formation.[63] Moreover, in a recent study of southwestern Melas Chasma, using high-resolution image, topographic and spectral datasets, eleven fan-shaped landforms were found. These fans add to growing evidence that Melas Chasma once held a lake which fluctuated in level.[64][65] A lake could have formed in the southwest part of Melas Chasma from runoff from local valley networks.[66][67]
Keith Harrison and Mary Chapman described strong evidence for a lake in the eastern part of Valles Marineris, especially in Coprates Chasma. It would have had an average depth of only 842 m—much shallower than the 5–10 km depth of parts of Valles Marineris. Still, its volume of 110,000 cubic kilometers would be comparable to Earth's Caspian Sea. The main evidence for such a lake is the presence of benches at the level that models show is where the lake level should be. Also, the low point in Eos Chasma where water would be expected to overflow is marked by fluvial features. The features look as if the flow came together in a small area and caused significant erosion.[68][69]
- Layers in the canyon wall in Coprates, as seen by Mars Global Surveyor, under the MOC Public Targeting Program.
Hellas Basin
The Hellas quadrangle contains part of the Hellas Basin, the largest known impact crater on the surface of Mars and the second largest in the Solar System. The depth of the crater is 7152 m[70] (23,000 ft) below the standard topographic datum of Mars. The basin is located in the southern highlands of Mars and is thought to have been formed about 3.9 billion years ago, during the Late Heavy Bombardment. It is believed that a giant lake existed in the Hellas Basin early in the planet's history with a possible depth of 5.5 km.[71][72] Possible shorelines have been discovered.[73][74] These shorelines are evident in alternating benches and scarps visible in Mars orbiting camera narrow-angle images. A good example of layers that were deposited in Hellas, and later exposed by erosion, is visible in Terby Crater on the north rim of Hellas. It used to be thought that Terby Crater contained a large delta.[75] However, newer observations have led researchers to think of the layered sequence as part of a group of layers that may have extended all the across Hellas. There is no valley at the northern rim of Terby large enough to have carried the large amount of sediments necessary to produce the layers.[71] Other observations argue against Terby containing a delta. In addition, Mars orbiting laser altimeter (MOLA) data show that the contacts of these sedimentary units mark contours of constant elevation for thousands of km, and in one case all around the basin.[76]
- Layers in Terby crater may have formed when the Hellas basin was filled with water.
- Northern part of Terby Crater showing many layers, as seen by CTX camera (on Mars Reconnaissance Orbiter). Parts of this image are enlarged in next two images.
- Northern part of Terby Crater showing many layers, as seen by CTX camera (on Mars Reconnaissance Orbiter).
- Northern part of Terby Crater showing many layers, as seen by CTX camera (on Mars Reconnaissance Orbiter).
Channels, believed to be formed by water, enter the basin on all sides.[77][78][79][80]
Dao Vallis begins near a large volcano, called Hadriaca Patera, so it is thought to have received water when hot magma melted huge amounts of ice in the frozen ground.[81] The partially circular depressions on the left side of the channel in the adjacent image suggest that groundwater sapping also contributed water.[82] The Hellas drainage basin may be almost one-fifth the area of the entire northern plains. A lake in Hellas in today's Martian climate would form thick ice at the top that would eventually be removed by sublimation: the ice would turn directly from a solid to a gas, as dry ice (solid CO2) does on Earth.[83] Glacial features (terminal moraines, drumlins, and eskers) have been found that may have been formed when the water froze.[81][84] A lake filling the Hellas Basin may have lasted a very long time, particularly if there were some geothermal sources of heat. Consequently, life may have developed here.[71]
Gale Crater

At 10:32 p.m. PDT on Aug. 5, 2012 (1:32 a.m. EDT on Aug. 6, 2012), the Mars Science Laboratory rover, Curiosity, landed on Mars at 4°30′S 137°24′E / 4.5°S 137.4°E, at the foot of a layered mountain inside Gale crater. The crater is named for Walter F. Gale (1865–1945), an amateur astronomer from Australia.
Gale is a crater on Mars near the northwestern part of the Aeolis quadrangle. Gale is 154 km (96 mi) in diameter and holds a mountain, Aeolis Mons (previously informally named "Mount Sharp" to pay tribute to geologist Robert P. Sharp) rising higher from the crater floor than Mount Rainier rises above Seattle. Gale is about the combined area of Connecticut and Rhode Island. Evidence exists that Gale Crater once held a large lake.[87][88][89] On August 6, 2012, the Mars Science Laboratory landed on Aeolis Palus near Aeolis Mons in Gale Crater.[88][89][90][91][92][93][94] The landing was 2.279 km (1.416 mi) from the target (4°35′31″S 137°26′25″E / 4.591817°S 137.440247°E), closer than any previous rover landing and well within the target area. As the days and months went on with Curiosity studying the surface, more and more discoveries and conclusions were released from NASA detailing the mounting evidence that Gale once contained a large lake. On September 27, 2012, NASA scientists announced that the Curiosity rover found evidence for an ancient streambed suggesting a "vigorous flow" of water on Mars.[95][96][97] On December 9, 2013, NASA reported that, based on evidence from Curiosity studying Aeolis Palus, Gale Crater contained an ancient freshwater lake which could have been a hospitable environment for microbial life.[98][99] Curiosity found fine-grained sedimentary rocks, which represent an ancient lake that would have been suited to support life based on chemolithoautotrophy. This liquid water environment possessed a neutral pH, low salinity, and iron and sulfur in forms usable to certain types of organisms. Carbon, hydrogen, oxygen, sulfur, nitrogen—the essential elements for life were measured. Gale’s ancient lake might have lasted hundreds to tens of thousands of years.[100][101]
Smectite clays (trioctahedral) that are formed in the presence of water were found by Curiosity in sedimentary rocks (mudstones) at Yellowknife Bay in Gale crater. The mudstone samples were named John Klein and Cumberland. They are believed to have formed later than the Noachian period which means that water may have existed there longer than previously thought.

Gale Crater contains a number of alluvial fans and deltas that provide information about lake levels in the past. These formations are: Pancake Delta, Western Delta, Farah Vallis delta and the Peace Vallis Fan.[102] In a press conference on December 8, 2014, Mars scientists discussed observations by Curiosity Rover that show Mars' Mount Sharp was built by sediments deposited in a large lake bed over tens of millions of years. This finding suggests the climate of ancient Mars could have produced long-lasting lakes at many places on the Planet. Rock layers indicate that a huge lake was filled and evaporated many times. The evidence was many deltas that were stacked upon each other.[103][104][105][106][107][108][109]
Gale Crater is considered to be a closed-basin lake, as channels lead into it, but none lead out.[26]
Minerals called clays and sulfates are byproducts of water. They also may preserve signs of past life. The history of water at Gale, as recorded in its rocks, is giving Curiosity lots of clues to study as it pieces together whether Mars ever could have been a habitat for small life forms called microbes. Gale is special because both clays and sulfate minerals, which formed in water under different conditions, can be observed.
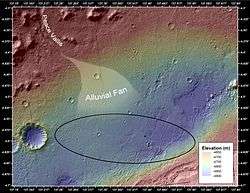
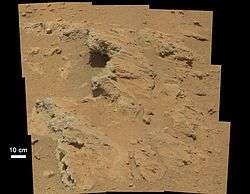
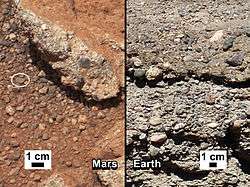
Holden Crater
Holden is a 140 km wide crater in the Margaritifer Sinus quadrangle. It is named after Edward Singleton Holden, an American astronomer, and the founder of the Astronomical Society of the Pacific.[110] Like some other craters on Mars, Holden has an outlet channel, Uzboi Vallis, that runs into it. Some features in the crater, especially lake deposits, seem to have been created by flowing water.[111] The crater's rim is cut with gullies, and at the end of some gullies are fan-shaped deposits of material transported by water.[111][112] The crater is of great interest to scientists because it has some of the best-exposed lake deposits.[113] One of the layers has been found by the Mars Reconnaissance Orbiter to contain clays.[63][111][114][115] Clays only form in the presence of water. It is believed that great amount of water went through this area; one flow was caused by a body of water larger than Earth's Lake Huron. This happened when water burst through a crater rim that was damming it.[116] Holden is an old crater, containing numerous smaller craters, many of which are filled with sediment. Indeed, over 150 m of sediment is exposed in Holden Crater, especially in southwestern part of the crater. The crater's central mountain is also obscured by sediment. Much of the sediment probably originated from river and lake deposits.[117] Holden Crater is in the Uzboi-Landon-Morava (ULM) outflow system.
Crater Holden based on THEMIS day-time image | |
Planet | Mars |
---|
![]() Uzboi Vallis based on THEMIS day-time image | |
Length | 366.0 |
---|---|
Naming |
Dry riverbed in Russia. |
- Close-up of Channels on Rim of Holden Crater, as seen by THEMIS. Image is located in the Margaritifer Sinus quadrangle.
Geologic history of Holden Crater
Studies of the whole region around Holden Crater have resulted in an understanding of a complex sequence of events that shaped the crater, which included two different lakes.[118] A large series of rivers called the Uzboi-Ladon-Morava (ULM) system drained water from the Argyre Basin, site of a large lake.[119][120][121] When an impact occurred and produced Holden Crater, the system was blocked by a crater rim almost a kilometer in height. Eventually water from drainage from the walls, with possibly a contribution from groundwater, collected to make the first lake.[71][122][123] This lake was deep and long lasting. The lowest level of sedimentary rocks was deposited in this lake. Much water was inbounded in Uzboi Vallis because the rim of Holden Crater blocked the flow. Some of the backed up water came from Nirgal Vallis which had a discharge of 4800 cubic meters/second.[124] At a certain point the stored water broke through the rim of Holden and created a second, shorter lived lake 200–250 m deep.[125] Water with a depth of at least 50 m entered Holden at a rate that 5-10 times the discharge of the Mississippi River.[126][127][128][129] Terraces and the presence of large rocks (tens of meters across) support these high discharge rates.[71][126][130][131][132]
Western Elysium Planitia Paleolake
There is evidence for a large lake in Western Elysium; however some researchers believe large lava flows can explain the terrain.[71][133] The basin of this supposed lake has an area of more than 150 km2 and is covered with fractured plates and sinuous ridges that look like pack-ice on the Earth.[134][135][136] Sorted patterned ground and erosion patterns in polygonal terrain in the region support ice-rich material; hence a lake. Also, the presence of streamlined islands, cataracts, and dendritic channel systems suggest formation by water from a lake.[137] Some surfaces here show "Rootless cones" which are mounds with pits. They can be caused by explosions of lava with ground ice when lava flows on top of ice-rich ground. The ice melts and turns into a vapor that expands in an explosion that produces a cone or ring. Features like these are found in Iceland, when lavas cover water-saturated substrates.[138][139][140] The western Elysium Planitia basin can be described as almost a perfect equipotential surface because it slopes only about 10 m over a 500 km distance—that’s about as level as the Earth’s ocean.[141] This very gentle slope argues against a lava flow.[142] In places, it has been found that the flow surface has been lowered by 50% which is expected if the flow was of water, but not if it were lava.[135] The maximum depth of the lake was estimated to be between 31 and 53 m.[135] The Western Elysium Paleolake is in the southern part of the Elysium quadrangle, south of the Elysium volcanic field and near Cerberus Fossae. It is believed that the water for this paleolake emerged from troughs in Cerberus Fossae. Several opinions have been advanced to explain the exact mechanism, including groundwater discharge and[143][144] a dike penetrating a cryosphere,[145]
- Troughs of the Cerberus Fossae group, as seen by HiRISE under the HiWish program.
- Portion of a trough (fossa) in Elysium, as seen by HiRISE under the HiWish program. Troughs are part of the Cerberus Fossae group.
Argyre basin
The Argyre basin was created by a giant impact that occurred 70 million years after the Hellas impact.[146] It is believed to have contained a lake early in the history of Mars.[147] The Argyre basin is in the Argyre quadrangle. At least three river valleys (Surius Vallis, Dzigal Vallis, and Palacopus Vallis) drain into it from the south. After the Argyre lake froze solid, the ice formed eskers which are visible today.[148][149] An article written by 22 researchers in Icarus concluded that the impact that formed the Argyre basin probably struck an ice cap or a thick permafrost layer. Energy from the impact melted the ice and formed a giant lake that eventually sent water to the North. The lake's volume was equal to that of Earth's Mediterranean Sea. The deepest part of the lake may have taken more than a hundred thousand years to freeze, but with the help of heat from the impact, geothermal heating, and dissolved solutes it may have had liquid water for many millions of years. Life may have developed in this time. This region shows a great deal of evidence of glacial activity with flow features, crevasse-like fractures, drumlines, eskers, tarns, aretes, cirques, horns, U-shaped valleys, and terraces. Because of the shapes of Argyre sinuous ridges, the authors concluded that they are eskers.[150]
- MOLA maps showing the geographic context of Argyre.
- MOLA map showing boundaries for Argyre Planitia and other regions
- Scene in Argyre quadrangle with gullies, alluvial fans, and hollows, as seen by HiRISE under HiWish program. Enlargements of parts of this image are below.
- Several levels of alluvial fans, as seen by HiRISE under HiWish program. Locations of these fans are indicated in the previous image. Fans are formed with the action of water.
- Small, well-formed alluvial fan, as seen by HiRISE under HiWish program. Location of this fan is shown in an image displayed above. Water is involved with fan formation.
Lakes in Valles Marineris
Map of Coprates quadrangle from Mars Orbiter Laser Altimeter (MOLA) data. The highest elevations are red and the lowest are blue. |
Over the years, it has been suggested that lakes of various sizes existed in the giant Valles Marineris.[151][152][153][154] However, the matter is still debated. Much discussion centers on the origin of layered structures called interior layered deposits (ILD’s). They are widely distributed in the Valles Marineris system. Some are free-standing mesas and mounds. Interior layered deposits are up to 9 km thick.[155]
Parts of the floors of Candor Chasma and Juventae Chasma contain interior layered deposits. These layers may have formed when the whole area was a giant lake. However, many other ideas have been advanced to explain them.[35] High-resolution structural and geologic mapping in west Candor Chasma, presented in March 2015, showed that the deposits on the floor of the Candor chasma are basin filling sediments that were deposited in a wet playa like setting; hence water was involved in their formation.[56]
One problem with the idea of large lakes in Valles Marineris is that there are no evident sources for the huge amount of water that would be required. Although many small channels exist in the region, there are no major channels. However, much water may have entered the system through the ground.[156][157] Although much controversy exists concerning a lake that filled the whole Valles Marineris system, there is a fair amount of acceptance over smaller lakes in the system.
Parts of the floors of Candor Chasma and Juventae Chasma contain interior layered deposits. These layers may have formed when the whole area was a giant lake. However, many other ideas have been advanced to explain them.[71] High-resolution structural and geologic mapping in west Candor Chasma, presented in March 2015, showed that the deposits on the floor of the Candor chasma are basin filling sediments that were deposited in a wet playa like setting; hence water was involved in their formation.[56]
Minerals that are usually formed in the presence of water have been discovered in interior layered deposits; thus giving strong support for lakes. Some ILD’s contain hydrated sulfate deposits. Sulfate formation involves the presence of water. The European Space Agency's Mars Express found possible evidence of the sulfates epsomite and kieserite.[57] Likewise, ferric oxides in the form of crystalline grey hematite that probably required water for its formation have been found.[35][58][158]
Ritchey Crater
Ritchey Crater is a crater in the Coprates quadrangle. It is 79 km in diameter and was named after George W. Ritchey, an American astronomer (1864–1945).[159] There is strong evidence that it was once a lake.[160][161] Ritchey Crater has been suggested as a landing site for a Mars Rover.[161] A thick sequence of sedimentary deposits that include clay is found in the crater.[160][162] Clay deposits indicate that water was probably present for a time. The presence of fluvial features along crater wall and rim, as well as alluvial/fluvial deposits, support the idea of lots of water being present at some time in the past.
- Western side of Ritchey Crater, as seen by CTX camera (on Mars Reconnaissance Orbiter).
- Fan along western wall of Ritchey Crater, as seen by CTX camera (on Mars Reconnaissance Orbiter). Note: this is an enlargement of previous image.
Jezero (crater)
![]() Jezero crater and region | |
Planet | Mars |
---|---|
Diameter | 49.0 km (30.4 mi) |
Eponym | Jezero, meaning "lake" in Slavic languages |
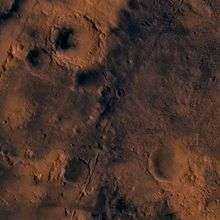
]
Jezero is a crater on Mars located at 18°51′18″N 77°31′08″E / 18.855°N 77.519°E[163] in the Syrtis Major quadrangle. The diameter of the crater is about 49.0 km (30.4 mi). Thought to have once been flooded with water, the crater contains a fan-delta deposit rich in clays.[164]

Jezero crater, once considered a site for the Mars Science Laboratory, is a proposed landing site for the Mars 2020 rover mission.[165] Clay minerals have been detected in and around the crater.[166][167][167][168] The Mars Reconnaissance Orbiter identified smectite clays.[169] The Mars Reconnaissance Orbiter identified smectite clays.[169] Clays form in the presence of water, so this area probably once held water and maybe life in ancient times. The surface in places are cracked into polygonal patterns. Such shapes often form when clay dries out.[163]
Researchers described in a paper, released in March 2015, how an ancient Martian lake system existed in Jezero Crater. The study advanced the idea that water filled the crater at least two separate times.[166][170][171][172] There are two channels on the northern and western sides of the crater that probably supplied it with water; both of these channels have delta-like deposit where sediment was carried by water and deposited in the lake.[173] Pictures show layers and meanders.[174]
A primary aim of the Mars 2020 mission is to search for signs of ancient life. It is hoped that a later mission could then return samples from sites identified as probably containing remains of life. To safely bring the craft down, a 12-mile wide, smooth, flat circular area is needed. Geologists hope to examine places where water once ponded.[175] They would like to examine sediment layers.
Eridania Lake
Eridania Lake is a theorized ancient lake with a surface area of roughly 1.1 million square kilometers.[176][177][178] It's maximum depth is 2,400 meter,s and its volume is 562,000 km2 Three basins make up the lake Ariadnes (centered at 175 E, 35 S), Atlantis (Centered at 182 E, 32 S), and Gorgonum (Centered at 192 E, 37 S).[179] It is located at the source of the Ma'adim Vallis outflow channel and extends into Eridania quadrangle and the Phaethontis quadrangle.[180][181] As Eridania Lake dried out in the late Noachian epoch it divided into a series of smaller lakes.[71][182][183][184] Clays which require water for their formation have been found within the borders of this supposed lake. They were identified as Mg/Fe-bearing phyllosilicates and Al-rich phyllosilicates, using with hyperspectral data from CRISM.[185] Further study, published in 2016, using both OMEGA (Visible and Infrared Mineralogical Mapping Spectrometer on Mars Express) and CRISM found that a capping layer lies above a Al-rich clay layer (probably Al-smectite and/or kaolins. Beneath this layer is Fe-rich clay, called nontronite smectite, and then a layer of zeolite or hydrated sulfate. Small deposits of alunite and jarosite were also discovered. The clay minerals provide favorable conditions for the preservation of past Martian life traces.[179]
Columbus crater
Columbus Crater is a crater in the Memnonia quadrangle, is 119 km in diameter, and was named after Christopher Columbus, Italian explorer (1451–1506).[110][186] Research with an orbiting near-infrared spectrometer, which reveals the types of minerals present based on the wavelengths of light they absorb, found evidence of layers of both clay and sulfates in Columbus crater. This is exactly what would appear if a large lake had slowly evaporated.[71][187][188][189] Moreover, because some layers contained gypsum, a sulfate which forms in relatively fresh water, life could have formed in the crater.[190] The CRISM instrument on the Mars Reconnaissance Orbiter found kaolinite, hydrated sulfates including alunite and possibly jarosite.[63] Further study concluded that gypsum, polyhydrated and monohydrated Mg/Fe-sulfates were common and small deposits of montmorillonite, Fe/Mg-phyllosilicates, and crystalline ferric oxide or hydroxide were found. Thermal emission spectra suggest that some minerals were in the tens of percent range. These minerals suggest that water was present in the crater.[188][191] Scientists are excited about finding hydrated minerals such as sulfates and clays on Mars because they are usually formed in the presence of water.[192] Places that contain clays and/or other hydrated minerals would be good places to look for evidence of life.[193] Sulfate minerals were found above aluminum-rich clays; this implies that early on, when the clays were formed, the water was more neutral and probably easier for life to develop. Sulfates are usually formed with more acid waters being present.[194]
![]() Columbus crater based on THEMIS day-time image | |
Planet | Mars |
---|---|
Diameter | 119 km |
Eponym | Christopher Columbus, Italian explorer (1451–1506) |
- Columbus Crater Layers, as seen by HiRISE. This false-color image is about 800 feet across. Some of the layers contain hydrated minerals.
![]() |
Wikimedia Commons has media related to Columbus (Martian crater). |
See also
- 2001 Mars Odyssey
- Alluvial fan
- Atmosphere of Mars
- Composition of Mars
- Curiosity (rover)
- Evidence of water on Mars found by Mars Reconnaissance Orbiter
- Geography of Mars
- Geological history of Mars
- Geology of Mars
- Groundwater on Mars
- Impact crater
- Life on Mars
- List of craters on Mars
- List of quadrangles on Mars
- List of valles on Mars
- Mariner 4
- Mars ocean hypothesis
- Mars Reconnaissance Orbiter
- Peace Vallis
- Phoenix (spacecraft)
- Ritchey (Martian crater)
- River delta
- Seasonal flows on warm Martian slopes
- Timeline of Mars Science Laboratory
- Uzboi-Landon-Morava (ULM)
- Water on Mars
References
- ↑ "Mariner 4: First Spacecraft to Mars". space.com. Retrieved 4 July 2015.
- ↑ "Blast from the past: Mariner 4's images of Mars | The Planetary Society". planetary.org. Retrieved 4 July 2015.
- ↑ Snyder, C., V. Moroz. 1992. Spacecraft exploration of Mars. In Kieffer, H., B. Jakosky, C. Snyder, M. Matthews, (eds). 1992. Mars. University of Arizona Press. Tucson.
- ↑ "What is the evidence for water on Mars?". astronomycafe.net. Retrieved 4 July 2015.
- ↑ Madeleine, J. et al. 2007. Mars: A proposed climatic scenario for northern mid-latitude glaciation. Lunar Planet. Sci. 38. Abstract 1778.
- ↑ Madeleine, J. et al. 2009. Amazonian northern mid-latitude glaciation on Mars: A proposed climate scenario. Icarus: 203. 300-405.
- ↑ Mischna, M. et al. 2003. On the orbital forcing of Martian water and CO2 cycles: A general circulation model study with simplified volatile schemes. J. Geophys. Res. 108. (E6). 5062.
- ↑ Newsom, H. 2010. Heated Lakes on Mars. In Cabrol, N. and E. Grin (eds.). 2010. Lakes on Mars. Elsevier. NY.
- ↑ Abramov, O.; Kring, D. (2005). "Impact-induced hydrothermal activity on early Mars". Journal of Geophysical Research. 110. Bibcode:2005JGRE..11012S09A. doi:10.1029/2005je002453.
- ↑ Newsom, H (1980). "Hydrothermal alteration of impact melt sheets with implications for Mars". Icarus. 44 (1): 207–216. Bibcode:1980Icar...44..207N. doi:10.1016/0019-1035(80)90066-4.
- ↑ Newsom, H.; et al. (1996). "Impact crater lakes on Mars". J. Geophys. Res. 101 (E6): 14951–9144955. Bibcode:1996JGR...10114951N. doi:10.1029/96je01139.
- ↑ McKay, C.; Davis, W. (1991). "Duration of liquid water habitats on early Mars". Icarus. 90 (2): 214–221. Bibcode:1991Icar...90..214M. doi:10.1016/0019-1035(91)90102-y.
- ↑ Bibring, J., et al. 2006. Global mineralogical and aqueous history derived from OMEGA observations. Science: 312, 400-404.
- ↑ Murchie, S., et al. 2008. First results from the Compact Reconnaissance Imaging Spectrometer for Mars (CRISM), LPSC XXXIX abstract 1472.
- ↑ Zolotov, M., M. Mironenko. 2008. Formation and fate of phyllosilicates on the surface of Mars: Geochemical modeling of aqueous weathering. LPSC XXXIX, Abstract 3365.
- ↑ Carr, M., J. Head. In Cabrol, N. and E. Grin (eds.). 2010. Lakes on Mars. Elsevier. NY
- ↑ Gendrin, A. et al. 2005. Sulfates in Martian Layered Terrains: The OMEGA/Mars express view. Science: 307, 1587–1591.
- ↑ "Evidence of Vast Quantities of Water Ice on Mars". universetoday.com. Retrieved 4 July 2015.
- ↑ "Lunar & Planetary Lab at The University of Arizona". web.archive.org. Retrieved 4 July 2015.
- ↑ "Ground ice on Mars is patchy and variable | Mars Odyssey Mission THEMIS". themis.asu.edu. Retrieved 4 July 2015.
- ↑ "Ice Under the Lander? | Phoenix on Mars". phoenixonmars.wordpress.com. Retrieved 4 July 2015.
- ↑ "Confirmation of Water on Mars". Phoenix Mars Lander. NASA. 20 June 2008. Archived from the original on 1 July 2008.
- ↑ De Hon, R. 1992. Martian lake basins and lacustrine plains. Earth Moon Planets: 56, 95-122.
- 1 2 Cabrol, N., E. Grin. 1999. Distribution, classification, and ages of martian impact crater lakes. Icarus: 142, 160-172.
- ↑ Cabrol, N., E. Grin. 2001. The evolution of lacustrine environments on Mars: Is Mars only hydrologically dormant. Icarus: 149, 291-328.
- 1 2 Goudge, T., K. Aureli, J. Head, C. Fassett, J. Mustard. 2015. Classification and analysis of candidate impact crater-hosted closed-basin lakes on Mars. Icarus: 260, 346-367.
- ↑ Fassett, C. J. Head. 2008. Valley network-fed, open-basin lakes on Mars: Distribution and implications for Noachian surface and subsurface hydrology. Icarus: 198, 37-56.
- ↑ Irwin, R. et al. 2005. An intense terminal epoch of widespread fluvial activity on early Mars. 2. Increased runoff and paleolake development. J. Geophys. Res. 10, E12S15 doi:10.1029/2005JE002460
- ↑ Fassett, C.; Head, J. (2008). "Valley network-fed, open-basin lakes on Mars: Distribution and implications for Noachian surface and subsurface hydrology". Icarus. 198: 37–56. Bibcode:2008Icar..198...37F. doi:10.1016/j.icarus.2008.06.016.
- ↑ Grant, J. T. Parker (2002). "Drainage evolution in the Margaritifer Sinus region, Mars". J. Geophys. Res. 107: 5066. Bibcode:2002JGRE..107.5066G. doi:10.1029/2001JE001678.
- ↑ Head, J., S. Pratt. 2001. Closed chaos basins on Mars: Evidence for regional groundwater drawdown and collapse. Lunar Planet. Sci. XXXII. Abstract 1774.
- ↑ Irwin, R.; et al. (2002). "A large paleolake basin at the head of Ma'adim Vallis, Mars". Science. 296: 2209–2212. doi:10.1126/science.1071143. PMID 12077414.
- ↑ Irwin, R.; et al. (2004). "Geomorphology of Ma'adim Vallis, Mars, and associated paleolake basins". J. Geophys. Res. 109: E12009. Bibcode:2004JGRE..10912009I. doi:10.1029/2004JE002287.
- 1 2 Brandenburg, John E. (1987), "The Paleo-Ocean of Mars", MECA Symposium on Mars: Evolution of its Climate and Atmosphere, Lunar and Planetary Institute, pp. 20–22, retrieved 19 February 2014
- 1 2 3 4 5 Cabrol, N. and E. Grin (eds.). 2010. Lakes on Mars. Elsevier. NY
- ↑ Clifford, S. M.; Parker, T. J. (2001). "The Evolution of the Martian Hydrosphere: Implications for the Fate of a Primordial Ocean and the Current State of the Northern Plains". Icarus. 154: 40–79. Bibcode:2001Icar..154...40C. doi:10.1006/icar.2001.6671.
- ↑ Baker, V. R.; Strom, R. G.; Gulick, V. C.; Kargel, J. S.; Komatsu, G.; Kale, V. S. (1991). "Ancient oceans, ice sheets and the hydrological cycle on Mars". Nature. 352: 589–594. Bibcode:1991Natur.352..589B. doi:10.1038/352589a0.
- ↑ "Mars: The planet that lost an ocean's worth of water". ScienceDaily. web.archive.org. Retrieved 4 July 2015.
- ↑ "Nasa finds evidence of a vast ancient ocean on Mars". msn.com. Retrieved 4 July 2015.
- ↑ Villanueva, G.; Mumma, M.; Novak, R.; Käufl, H.; Hartogh, P.; Encrenaz, T.; Tokunaga, A.; Khayat, A.; Smith, M. (2015). "Strong water isotopic anomalies in the martian atmosphere: Probing current and ancient reservoirs". Science. 348: 218–221. doi:10.1126/science.aaa3630.
- 1 2 Read, Peter L. and S. R. Lewis, "The Martian Climate Revisited: Atmosphere and Environment of a Desert Planet", Praxis, Chichester, UK, 2004.
- ↑ Staff (13 June 2007). "Mars Probably Once Had A Huge Ocean". University of California - Berkeley. Science Daily. Retrieved 19 February 2014.
- ↑ Staff (26 January 2001). "Mars Ocean Hypothesis Hits the Shore". Astrobiology Magazine. Retrieved 19 February 2004.
- ↑ Malin, M. C., and Edgett, K. S., 1999. Oceans or Seas in the Martian Northern Lowlands: High Resolution Imaging Tests of Proposed Coastlines, Geophys. Res. Letters, V. 26, No. 19, p. 3049–3052
- ↑ Staff (26 November 2009). "Martian North Once Covered by Ocean". Astrobiology Magazine. Retrieved 19 February 2014.
- ↑ Staff (23 November 2009). "New Map Bolsters Case for Ancient Ocean on Mars". Space.com. Retrieved 19 February 2014.
- ↑ DiAchille, G; Hynek, B. (2010). "Ancient ocean on Mars supported by global distribution of deltas and valleys". Nat. Geosci. 3: 459–463. Bibcode:2010NatGe...3..459D. doi:10.1038/ngeo891.
- ↑ DiBiasse; Limaye, A.; Scheingross, J.; Fischer, W.; Lamb, M. (2013). "Deltic deposits at Aeolis Dorsa: Sedimentary evidence for a standing body of water on the northern plains of Mars". Journal of Geophysical Research: Planets. 118: 1285–1302. doi:10.1002/jgre.20100.
- ↑ Mouginot, J.; Pommerol, A.; Beck, P.; Kofman, W.; Clifford, S. (2012). "Dielectric map of the Martian northern hemisphere and the nature of plain filling materials". Geophysical Research Letters. 39: L02202. Bibcode:2012GeoRL..39.2202M. doi:10.1029/2011GL050286.
- ↑ Villanueva, G. L.; Mumma, M. J.; Novak, R. E.; Käufl, H. U.; Hartogh, P.; Encrenaz, T.; Tokunaga, A.; Khayat, A.; Smith, M. D. (2015). "Strong water isotopic anomalies in the martian atmosphere: Probing current and ancient reservoirs". Science. 348: 218–221. doi:10.1126/science.aaa3630.
- ↑ Webster, C.R.; et al. (2013). "Isotope Ratios of H, C, and O in CO2 and H2O of the Martian Atmosphere". Science. 341: 260–263. doi:10.1126/science.1237961. PMID 23869013.
- ↑ McCauley, J. 1978. Geologic map of the Coprates quadrangle of Mars. U.S. Geol. Misc. Inv. Map I-897
- ↑ Nedell, S.; et al. (1987). "Origin and evolution of the layered deposits in the Valles Marineris, Mars". Icarus. 70: 409–441. Bibcode:1987Icar...70..409N. doi:10.1016/0019-1035(87)90086-8.
- ↑ Weitz, C. and T. Parker. 2000. New evidence that the Valles Marineris interior deposits formed in standing bodies of water. LPSC XXXI. Abstract 1693
- ↑ Cabrol, N. and E. Grin (Eds.). 2010. Lakes on Mars. Elsevier. NY
- 1 2 3 Okubo, C. 2015. HIGH-RESOLUTION STRUCTURAL AND GEOLOGIC MAPPING IN CANDOR CHASMA. 46th Lunar and Planetary Science Conference. 1210.pdf
- 1 2 "Bath Salts in Candor Chasma? | Mars Odyssey Mission THEMIS". Themis.asu.edu. Retrieved 18 August 2012.
- 1 2 Christensen, P.; et al. (2001). "Global mapping of Martian hematite mineral deposits: Remnants of water-driven processes on early Mars". J. Geophys. Res. 106: 23873–23885. Bibcode:2001JGR...10623873C. doi:10.1029/2000je001415.
- ↑ Weitz, C.; et al. (2008). "Gray hematite distribution and formation in Ophir and Candor Chasmata". J. Geophys. Res. 113: E02016. Bibcode:2008JGRE..113.2016W. doi:10.1029/2007je002930.
- ↑ Cattermole, Peter John (2001). Mars: the mystery unfolds. Oxford University Press. p. 105. ISBN 0-19-521726-8.
- ↑ "HiRISE | Eroding Layers in Melas Chasma (PSP_004054_1675)". hirise.lpl.arizona.edu. Retrieved 4 July 2015.
- ↑ "HiRISE | MSL Landing Site in Melas Chasma (PSP_002828_1700)". hirise.lpl.arizona.edu. Retrieved 4 July 2015.
- 1 2 3 Murchie, S. et al. 2009. A synthesis of Martian aqueous mineralogy after 1 Mars year of observations from the Mars Reconnaissance Orbiter. Journal of Geophysical Research: 114.
- ↑ Williams, R. and C. Weitz. Reconstructing the aqueous history within the southwestern Melas basin, Mars: Clues from stratigraphic and morphometric analyses of fans. Icarus:242, 19-37.
- ↑ Davis, J., P. Grindrod, R. Williams, S. Gupta, M. Balme. 2015. STRATIGRAPHIC EVIDENCE OF EPISODIC FLUVIAL ACTIVITY IN THE SOUTH MELAS CHASMA BASIN, VALLES MARINERIS, MARS. 46th Lunar and Planetary Science Conference. 1932.pdf
- ↑ Quantin, et al. 2005.
- ↑ Metx, et al. 2009.
- ↑ Harrison, K., M. Chapman. 2010. Episodic ponding and outburst flooding associated with chaotic terrains in Valles Marineris In Cabrol, N. and E. Grin (eds.). 2010. Lakes on Mars. Elsevier. NY.
- ↑ Harrison, K., M. Chapman. 2008. Evidence for ponding and catastrophic floods in central Valles Marineris, Mars. Icarus: 198, 351-364.
- ↑ Martian Weather Observation MGS radio science measured 11.50 mbar at 34.4° S 59.6° E -7152 meters.
- 1 2 3 4 5 6 7 8 9 Cabrol, N. and E. Grin (eds.). 2010. Lakes on Mars. Elsevier. NY.
- ↑ Voelker, M., et al. 2016. DISTRIBUTION AND EVOLUTION OF LACUSTRINE AND FLUVIAL FEATURES IN HELLAS PLANITIA, MARS, BASED ON PRELIMINARY RESULTS OF GRID-MAPPING. 47th Lunar and Planetary Science Conference (2016) 1228.pdf.
- ↑ Crown, D., et al. 2005. Styles and timing of volatile-driven activity in the eastern Hellas region of Mars" J. Geophys. Res. 102, E112S122 doi:10.1029/2005JE002496
- ↑ Moore, J.; Wilhelms, D. (2001). "Hellas as a possible site of ancient ice-covered lakes on Mars". Icarus. 154 (2): 258–276. Bibcode:2001Icar..154..258M. doi:10.1006/icar.2001.6736.
- ↑ Ansan, V. et al. 2005. Analysis of layered deposits in Terby crater Hellas region, Mars using multiple datasets MOC, THEMIS, and OMEGA/MEX date. Lunar Planet. Sci., XXXVI (CD-ROM). Abstract 1324.
- ↑ Wilson, S., et al. 2010. Evidence for ancient lakes in the Hellas region. In Cabrol, N. and E. Grin (eds.). 2010. Lakes on Mars. Elsevier. NY.
- ↑ Carr, M.; Chung, F. (1997). "Martian drainage densities". J. Geophys. Res. 102: 9145–9152. Bibcode:1997JGR...102.9145C. doi:10.1029/97je00113.
- ↑ Greeley, R., J. Guest. 1987. Geologic map of the eastern equatorial region of Mars. U.S. Geological Survey Miscellaneous Investigations Series I-1802-B, scale 1:15,000,000
- ↑ Leonard, G., K. Tanaka. 2001. Geologic map of the Hellas region of Mars, U.S. Geological Survey Miscellaneous Investigations Series I-2694, scale 1:5,000,000
- ↑ Tanaka, K., G. Leonard. 1995. Geology and landscape of the Hellas region of Mars, J. Geophys. Res. 100 (E3), 5407_5432
- 1 2 Carr, Michael H. (2006). The Surface of Mars. Cambridge University Press. p. . ISBN 978-0-521-87201-0.
- ↑ "Dao Vallis | Mars Odyssey Mission THEMIS". themis.asu.edu. Retrieved 4 July 2015.
- ↑ Moore, J; Wilhelms, Don E. (2001). "Hellas as a possible site of ancient ice-covered lakes on Mars". Icarus. 154 (2): 258–276. Bibcode:2001Icar..154..258M. doi:10.1006/icar.2001.6736.
- ↑ Kargel, J.; Strom, R. (1991). "Terrestrial glacial eskers: analogs for martian sinuous ridges" (PDF). LPSC. XXII: 683–684. Bibcode:1991LPI....22..683K.
- ↑ "Ice Sculptures Fill The Deepest Parts of Mars". universetoday.com. Retrieved 12 July 2015.
- ↑ "Mega-scale civil engineering on Mars". forum.nasaspaceflight.com. Retrieved 12 July 2015.
- ↑ Staff (October 8, 2015). "Wet Paleoclimate of Mars Revealed by Ancient Lakes at Gale Crater". Astrobiology web. Retrieved October 9, 2015.
- 1 2 Clavin, Whitney (October 8, 2015). "NASA's Curiosity Rover Team Confirms Ancient Lakes on Mars". NASA. Retrieved October 9, 2015.
- 1 2 Grotzinger, J.P.; et al. (October 9, 2015). "Deposition, exhumation, and paleoclimate of an ancient lake deposit, Gale crater, Mars". Science. 350 (6257): aac7575. doi:10.1126/science.aac7575. Retrieved October 9, 2015.
- ↑ NASA Staff (10 August 2012). "Curiosity's Quad - IMAGE". NASA. Retrieved 11 August 2012.
- ↑ Agle, D. C. (28 March 2012). "'Mount Sharp' On Mars Links Geology's Past and Future". NASA. Retrieved 31 March 2012.
- ↑ Staff (29 March 2012). "NASA's New Mars Rover Will Explore Towering 'Mount Sharp'". Space.com. Retrieved 30 March 2012.
- ↑ http://dsc.discovery.com/news/2008/11/21/mars-landing-sites-02.html
- ↑ http://www.space.com/missionlaunches/mars-science-laboratory-curiosity-landing-sites-100615.htm
- 1 2 Brown, Dwayne; Cole, Steve; Webster, Guy; Agle, D.C. (27 September 2012). "NASA Rover Finds Old Streambed On Martian Surface". NASA. Retrieved 28 September 2012.
- 1 2 NASA (27 September 2012). "NASA's Curiosity Rover Finds Old Streambed on Mars - video (51:40)". NASAtelevision. Retrieved 28 September 2012.
- 1 2 Chang, Alicia (27 September 2012). "Mars rover Curiosity finds signs of ancient stream". Associated Press. Retrieved 27 September 2012.
- ↑ Chang, Kenneth (9 December 2013). "On Mars, an Ancient Lake and Perhaps Life". New York Times. Retrieved 9 December 2013.
- ↑ Various (9 December 2013). "Science - Special Collection - Curiosity Rover on Mars". Science. Retrieved 9 December 2013.
- ↑ "A Habitable Fluvio-Lacustrine Environment at Yellowknife Bay, Gale Crater, Mars". sciencemag.org. Retrieved 4 July 2015.
- ↑ Grotzinger, J.; et al. ". 2014. A Habitable Fluvio-Lacustrine Environment at Yellowknife Bay, Gale Crater, Mars". Science. doi:10.1126/science.124277.
- ↑ Dietrich, W., M. Palucis, T. Parker, D. Rubin, K.Lewis, D. Sumner, R. Williams. 2014. Clues to the relative timing of lakes in Gale Crater. Eighth International Conference on Mars (2014) 1178.pdf.
- ↑ Brown, Dwayne; Webster, Guy (8 December 2014). "Release 14-326 - NASA's Curiosity Rover Finds Clues to How Water Helped Shape Martian Landscape". NASA. Retrieved 8 December 2014.
- ↑ Kaufmann, Marc (8 December 2014). "(Stronger) Signs of Life on Mars". New York Times. Retrieved 8 December 2014.
- ↑ "NASA's Curiosity rover finds clues to how water helped shape Martian landscape -- ScienceDaily". web.archive.org. Retrieved 4 July 2015.
- ↑ "JPL | Videos | The Making of Mount Sharp". jpl.nasa.gov. Retrieved 4 July 2015.
- ↑ "JPL | News | NASA's Curiosity Rover Finds Clues to How Water Helped Shape Martian Landscape". jpl.nasa.gov. Retrieved 4 July 2015.
- ↑ "Martian fluvial conglomerates at Gale Crater". pubs.er.usgs.gov. Retrieved 4 July 2015.
- ↑ Williams, R.; et al. (2013). "Martian fluvial conglomerates at Gale Crater". Science. 340: 1068–1072. doi:10.1126/science.1237317.
- 1 2 "Google Mars". google.com. Retrieved 4 July 2015.
- 1 2 3 "The 4th MSL Landing Site Workshop: Day 2 - Holden Crater - Martian Chronicles - AGU Blogosphere". blogs.agu.org. Retrieved 4 July 2015.
- ↑ Moore, J. A. Howard. 2005. Large alluvial fans on Mars. Journal of Geophysical Research: 110, E04005.
- ↑ "Holden Crater: Where Waters Ran | Mars Odyssey Mission THEMIS". themis.asu.edu. Retrieved 12 July 2015.
- ↑ Grotzinger, J. and R. Milliken (eds.) 2012. Sedimentary Geology of Mars. SEPM
- ↑ "HiRISE | Proposed MSL Landing Site in Holden Crater (PSP_008193_1535)". hirise.lpl.arizona.edu. Retrieved 12 July 2015.
- ↑ "HiRISE | Megabreccia at Holden Crater (PSP_003077_1530)". hirise.lpl.arizona.edu. Retrieved 4 July 2015.
- ↑ Grant, J., R. Irwin, S. Wilson. 2010. Aqueous depositional settings in Holden crater, Mars. In Cabrol, N. and E. Grin (eds.). 2010. Lakes on Mars. Elsevier. NY
- ↑ Grant, J. 1987. The geomorphic evolution of Eastern Margaritifer Sinus, Mars. Adv. Planet. Geol. NASA Tech memo. 89889871, 1-268.
- ↑ Baker, V. 1982. The Channels of Mars. University of Texas Press, Austin, TX.
- ↑ Philillips, R. et al. 2001. Ancient geodynamics and global-scale hydrology on Mars. Science: 291, 2587_2591.
- ↑ Saunders, S. 1979. Geologic map of the Margaritifer Sinus quadrangle of Mars, U.S. Geol. Surv. Misc. Invest. Ser. Map I-1144, scale 1:5M.
- ↑ Malin, M., K. Edgett. 2000. Evidence for recent groundwater seepage and surface runoff on Mars. Science: 302, 1931–1934.
- ↑ Moore, J.; Howard, A. (2005). "Large alluvial fans on Mars". J. Geophys. Res. 110: E04005. doi:10.1029/2005JE002352.
- ↑ Irwin, J.; Craddock, R.; Howard, R. (2005). "Interior channels in Martian valley networks: Discharge and runoff production". Geology. 33: 489–492. doi:10.1130/g21333.1.
- ↑ Grant, J., R. Irwin, S. Wilson. 2010. Aqueous depositional settings in Holden crater, Mars In Cabrol, N. and E. Grin (eds.). 2010. Lakes on Mars. Elsevier. NY.
- 1 2 Grant, J.; Parker, T. (2002). "Drainage evolution of the Margaritifer Sinus region, Mars". J. Geophysic. Res. 107. Bibcode:2002JGRE..107.5066G. doi:10.1029/2001JE001678.
- ↑ Komar, P (1979). "Comparisons of the hydraulics of water flows in Martian outflow channels with flows of similar scale on Earth". Icarus. 37: 156–181. Bibcode:1979Icar...37..156K. doi:10.1016/0019-1035(79)90123-4.
- ↑ Grant, J.; et al. (2008). "HiRISE imaging of impact megabreccia and sub-meter aqueous strata in Holden Crater, Mars". Geology. 36: 195–198. doi:10.1130/g24340a.1.
- ↑ Irwin; et al. (2005). "An intense terminal epoch of widespread fluvial activity on early Mars: 2. Increased runoff and paleolake development". J. Geophysical. Res. 110: E12S14. Bibcode:2005JGRE..11012S15I. doi:10.1029/2005JE002460.
- ↑ Boothroyd, J (1983). "Fluvial drainage systems in the Ladon Basin area: Margaritifer Sinus area, Mars". Geol. Soc. Am. Abstr. Programs. 15: 530.
- ↑ Grant, J. 1987. The geomorphic evolution of Eastern Margaritifer Sinus, Mars. Adv. Planet. Geol. NASA Tech memo. 89871, 1-268.
- ↑ Parker, T. 1985. Geomorphology and geology of the southwestern Margaritifer Sinus-northern Argyre region of Mars, California State University, M. S. Thesis, Los Angeles, California
- ↑ Jaeger, W., et al. 2008. Emplacement of Athabasca Vallis flood lavas, Lunar Plan. Sci. Conf. XXIX (CDROM). Abstr. # 1836.
- ↑ Brackenridge, G. 1993. Modern shelf ice, equatorial Aeolis quadrangle, Mars. Lunar Plan. Sci. Conf. XXIV. Abstr. # 175.
- 1 2 3 Murray, J.; et al. (2005). "Evidence from the Mars Express high resolution stereo camera for a frozen sea close to Mars' equator". Nature. 434: 352–355. doi:10.1038/nature03379. PMID 15772653.
- ↑ Rice, J. et al. 2002. Morphology of fresh outflow channel deposits on Mars. Lunar Plan. Sci. Conf. XXXIII (CDROM). Abstr. #2026.
- ↑ Balme, M. et al. 2010. The Western Elysium Planitia Palelake. In Cabrol, N. and E. Grin (eds.). 2010. Lakes on Mars. Elsevier. NY.
- ↑ Fagents, A.; Lanagan, P.; Greeley, R. (2002). "Rootless cones on Mars: a consequence of lava-ground ice interaction". Geological Society, London. 202: 295–317. doi:10.1144/gsl.sp.2002.202.01.15.
- ↑ "PSR Discoveries: Rootless cones on Mars". psrd.hawaii.edu. Retrieved 4 July 2015.
- ↑ Jaeger, W., L. Keszthelyi, A. McEwen, C. Dundas, P. Russell, and the HiRISE team. 2007. EARLY HiRISE OBSERVATIONS OF RING/MOUND LANDFORMS IN ATHABASCA VALLES, MARS. Lunar and Planetary Science XXXVIII 1955.pdf.
- ↑ Reigber, C. et al. 2007. A High Resolution Global Gravity Field Model Combining CHAMP and GRACE Satellite Mission and Surface Data: EIGEN-CG01C. GeoForschungsZentrum, Potsdam. Scientific Technical Report STR 06/07.
- ↑ Keszthelyi, L.; et al. (2006). "Flood lavas on Earth, Io, and Mars". J. Geol. Soc. London. 163: 253–364. doi:10.1144/0016-764904-503.
- ↑ Manga, M (2004). "Martian floods at Cerberus Fossae can be produced by groundwater". Geophys. Res. Lett. 31: L0202702. Bibcode:2004GeoRL..31.2702M. doi:10.1029/2003GL018958.
- ↑ Plescia, J (2003). "Cerberus Fossae, Elysium, Mars: a source for lava and water". Icarus. 164: 465–490.
- ↑ Head, J.; et al. (2003). "Generation of recent massive water floods at Cerberus Fossae, Mars by dike emplacement, cryospheric cracking and confined aquifer groundwater release". Geophys. Res. Lett. 30: 11. Bibcode:2003GeoRL..30.1577H. doi:10.1029/2003GL017135.
- ↑ Robbins; et al. (2013). "Large impact crater histories of Mars: The effect of different model crater age techniques". Icarus. 225: 173–184. Bibcode:2013Icar..225..173R. doi:10.1016/j.icarus.2013.03.019.
- ↑ Parker, T.; et al. (2000). "Argyre Planitia and the Mars global hydrological cycle". LPSC. XXXI: 2033.
- ↑ Kargel, J.; Strom, R. (1991). "Terrestrial glacial eskers: analogs for martian sinuous ridges". LPSC. XXII: 683–684.
- ↑ Michael H. Carr (2006). The surface of Mars. Cambridge University Press. ISBN 978-0-521-87201-0. Retrieved 21 March 2011.
- ↑ Dohm, J.; Hare, T.; Robbins, S.; Williams, J.-P.; Soare, R.; El-Maarry, M.; Conway, S.; Buczkowski, D.; Kargel, J.; Banks, M.; Fairén, A.; Schulze-Makuch, D.; Komatsu, G.; Miyamoto, H.; Anderson, R.; Davila, A.; Mahaney, W.; Fink, W.; Cleaves, H.; Yan, J.; Hynek, B.; Maruyama, S. (2015). "Geological and hydrological histories of the Argyre province, Mars". Icarus. 253: 66–98. Bibcode:2015Icar..253...66D. doi:10.1016/j.icarus.2015.02.017.
- ↑ Sharp, R (1973). "Mars: troughed terrain". J. Geophys. Res. 78: 4063–4072. Bibcode:1973JGR....78.4063S. doi:10.1029/jb078i020p04063.
- ↑ Lucchitta, B (1987). "Valles Marineris, Mars: wet debris flows and ground ice". Icarus. 72: 411–429. Bibcode:1987Icar...72..411L. doi:10.1016/0019-1035(87)90183-7.
- ↑ Lucchitta, B. 2008. HiRISE images of layered deposits in west Candor Chasma, Mars (I): wall rock relations, enigmatic ridges, and possible dikes. 39th Lunar Planet. Sci. Conf. Abstract 2169.
- ↑ Lucchitta, B. 2010. Lakes in Valles Marineris. In Cabrol, N. and E. Grin (eds.). 2010. Lakes on Mars. Elsevier. NY.
- ↑ Lucchitta, B.; et al. (1994). "Topography of Valles Marineris: implications for erosional and structural history". J. Geophys. Res. 99: 3783–3798. Bibcode:1994JGR....99.3783L. doi:10.1029/93je03095.
- ↑ Andrews-Hanna; et al. (2007). "Meridiani Planum and the global 600 hydrology of Mars". Nature. 446: 163–168. doi:10.1038/nature05594. PMID 17344848.
- ↑ Murchie, S., et al. 2009. Evidence for the origin of layered deposits in Candor Chasma, Mars, from mineral composition and hydrologic modeling" J. Geophys. Res. 114, E00D05
- ↑ Weitz, C.; et al. (2008). "Gray hematite distribution and formation in Ophir and Candor Chasmata". J. Geophys. Res. 113: E02016. Bibcode:2008JGRE..113.2016W. doi:10.1029/2007je002930.
- ↑ "Planetary Names: Welcome". planetarynames.wr.usgs.gov. Retrieved 4 July 2015.
- 1 2 Sun, V.; Milliken, R. (2014). "The geology and mineralogy of Ritchey crater, Mars: Evidence for post-Noachian clay formation". Journal of Geophysical Research. 119 (4): 810–836. doi:10.1002/2013je004602.
- 1 2 Ralph Milliken (24 October 2007). "Clay Minerals in Water-Lain Sedimentary Deposits in the Southern Highlands: Evaluating Habitability on Mars with MSL" (PDF). Retrieved 12 July 2015.
- ↑ Milliken, R., et al. 2010. The case for mixed-layered clays on Mars, Lunar Planet. Sci. XLI, Abstract 2030
- 1 2 Wray, James (6 June 2008). "Channel into Jezero Crater Delta". NASA. Retrieved 6 March 2015.
- ↑ "Prime landing sites chosen for biggest Martian rover - space - 02 November 2007 - New Scientist". web.archive.org. Retrieved 12 July 2015.
- ↑ Staff (4 March 2015). "PIA19303: A Possible Landing Site for the 2020 Mission: Jezero Crater". NASA. Retrieved 7 March 2015.
- 1 2 Bibring, J.; et al. (2006). "Global mineralogical and aqueous Mars history derived from OMEGA/Mars Express data". Science. 312: 400–404. doi:10.1126/science.1122659. PMID 16627738.
- 1 2 Mangold, N.; et al. (2007). "Mineralogy of the Nili Fossae region with OMEGA/Mars Express data: 2. Aqueous alteration of the crust". Journal of Geophysical Research. 112: E08S04. Bibcode:2007JGRE..112.8S04M. doi:10.1029/2006JE002835.
- ↑ Poulet, F.; et al. (2005). "Phyllosilicates on Mars and implications for early martian climate". Nature. 438: 623–627. doi:10.1038/nature04274.
- 1 2 Murchie, S.; et al. (2009). "A synthesis of Martian aqueous mineralogy after 1 Mars year of observations from the Mars Reconnaissance Orbiter". Journal of Geophysical Research. 114 (E2). Bibcode:2009JGRE..114.0D06M. doi:10.1029/2009JE003342. Retrieved 7 March 2015.
- ↑ "Ancient Martian lake system records two water-related events | News from Brown". news.brown.edu. Retrieved 12 July 2015.
- ↑ "releases/2015/03/150325210744". sciencedaily.com. Retrieved 12 July 2015.
- ↑ Goudge, T.; et al. (2015). "Assessing the mineralogy of the watershed and fan deposits of the Jezero crater paleolake system, Mars". Journal of Geophysical Research. 120: 775–808. doi:10.1002/2014je004782.
- ↑ "Ancient Martian Lake System Records Two Water-related Events - SpaceRef". spaceref.com. Retrieved 12 July 2015.
- ↑ "JezeroCrater Lake: Phyllosilicate-bearing sediments from a Noachian valley network as a potential MSL landing site" (PDF). 22 October 2007. Retrieved 12 July 2015.
- ↑ Staff (2010). "The Floods of Iani Chaos". NASA. Retrieved 7 March 2015.
- ↑ Parker, T., D. Curie. 2001. Geomorphology 37. 303-328.
- ↑ de Pablo, M., M. Druet. 2002. XXXIII LPSC. Abstract #1032.
- ↑ de Pablo, M. 2003. VI Mars Conference, Abstract #3037.
- 1 2 Pajola, M., et al. 2016. Eridania Basin: An ancient paleolake floor as the next landing site for the Mars 2020 rover. Icauus: 275, 163-1823.
- ↑ Irwin, R.P.; et al. (2004). "Geomorphology of Maadim Vallis, Mars, and associated paleolake basins". Journal of Geophysical Research. 109: E12009. Bibcode:2004JGRE..10912009I. doi:10.1029/2004JE002287.
- ↑ Rossman, P. Irwin III; Ted A. Maxwell; Alan D. Howard; Robert A. Craddock; David W. Leverington (21 June 2002). "A Large Paleolake Basin at the Head of Ma'adim Vallis, Mars". Science. 296 (5576): 2209–2212. Bibcode:2002Sci...296.2209I. doi:10.1126/science.1071143. PMID 12077414.
- ↑ de Pablo, M. A.; Fairén, A. G.; Márquez, A. (3 March 2004). "The Geology of Atlantis Basin, Mars, and Its Astrobiological Interest" (PDF). 35th Lunar and Planetary Science Conference, 15–19 March 2004, League City, Texas. abstract no.1223.
- ↑ Rossman, R.; et al. (2002). "A large paleolake basin at the head of Ma'adim Vallis, Mars". Science. 296: 2209–2212. doi:10.1126/science.1071143. PMID 12077414.
- ↑ "HiRISE | Chaos in Eridania Basin (ESP_037142_1430)". uahirise.org. Retrieved 4 July 2015.
- ↑ Wendt, L.; Bishop, J.; Neukum, G. (2013). "Knob fields in the Terra Cimmeria/Terra Sirenum region of Mars: Stratigraphy, mineralogy and morphology". Icarus. 225: 200–2105. Bibcode:2013Icar..225..200W. doi:10.1016/j.icarus.2013.03.020.
- ↑ "Gazetteer of Planetary Nomenclature | Columbus". usgs.gov. International Astronomical Union. Retrieved 4 March 2015.
- ↑ "Sulfates and Clays in Columbus Crater, Mars | NASA". nasa.gov. Retrieved 4 July 2015.
- 1 2 Wray, J.; Milliken, R.; Dundas, C.; Swayze, G.; Andrews-Hanna, J.; Baldridge, A.; Chojnacki, M.; Bishop, J.; Ehlmann, B.; Murchie, S.; Clark, R.; Seelos, F.; Tornabene, L.; Squyres, S. (2011). "Columbus crater and other possible groundwater-fed paleolakes of Terra Sirenum, Mars". Journal of Geophysical Research: Planets. 116. Bibcode:2011JGRE..116.1001W. doi:10.1029/2010JE003694.
- ↑ Wray, J.; et al. (2009). "Columbus Crater and other possible plaeolakes in Terra Sirenum, Mars". Lunar and Planetary Science Conference. 40: 1896.
- ↑ "Martian "Lake Michigan" Filled Crater, Minerals Hint". news.nationalgeographic.com. Retrieved 4 July 2015.
- ↑ "Columbus crater and other possible groundwater-fed paleolakes of Terra Sirenum, Mars". pubs.er.usgs.gov. Retrieved 4 July 2015.
- ↑ "Target Zone: Nilosyrtis? | Mars Odyssey Mission THEMIS". themis.asu.edu. Retrieved 4 July 2015.
- ↑ "HiRISE | Craters and Valleys in the Elysium Fossae (PSP_004046_2080)". hirise.lpl.arizona.edu. Retrieved 4 July 2015.
- ↑ "Sulfates and Clays in Columbus Crater, Mars | NASA". nasa.gov. Retrieved 4 July 2015.
External links
- -- Lakes, Fans, Deltas and Streams: Geomorphic Constraints ...
- Lakes on Mars - Nathalie Cabrol (SETI Talks)
- Martian Ice - Jim Secosky - 16th Annual International Mars Society Convention