Calcium in biology
Calcium ions (Ca2+) play a vital role in the physiology and biochemistry of organisms and the cell. They play an important role in signal transduction pathways,[1][2] where they act as a second messenger, in neurotransmitter release from neurons, in contraction of all muscle cell types, and in fertilization. Many enzymes require calcium ions as a cofactor, those of the blood-clotting cascade being notable examples. Extracellular calcium is also important for maintaining the potential difference across excitable cell membranes, as well as proper bone formation.
Calcium levels in mammals are tightly regulated,[1][2] with bone acting as the major mineral storage site. Calcium ions, Ca2+, are released from bone into the bloodstream under controlled conditions. Calcium is transported through the bloodstream as dissolved ions or bound to proteins such as serum albumin. Parathyroid hormone secreted by the parathyroid gland regulates the resorption of Ca2+ from bone, reabsorption in the kidney back into circulation, and increases in the activation of vitamin D3 to Calcitriol. Calcitriol, the active form of vitamin D3, promotes absorption of calcium from the intestines and the mobilization of calcium ions from bone matrix. Calcitonin secreted from the parafollicular cells of the thyroid gland also affects calcium levels by opposing parathyroid hormone; however, its physiological significance in humans is dubious.
Calcium storages are intracellular organelles, that constantly accumulate Ca2+ ions and release them during certain cellular events. Intracellular Ca2+ storages include mitochondria and the endoplasmic reticulum.[3]
Animals
Vertebrates
In vertebrates, calcium ions, like many other ions, are of such vital importance to many physiological processes that its concentration is maintained within specific limits to ensure adequate homeostasis. This is evidenced by human plasma calcium, which is one of the most closely regulated physiological variables in the human body. Normal plasma levels vary between 1 and 2% over any given time. Approximately half of all ionized calcium circulates in its unbound form, with the other half being complexed with plasma proteins such as albumin, as well as anions including bicarbonate, citrate, phosphate, and sulfate.[4]
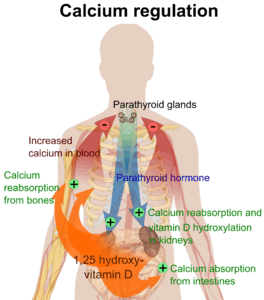
Different tissues contain calcium in different concentrations. For instance, Ca2+ (mostly calcium phosphate and some calcium sulfate) is the most important (and specific) element of bone and calcified cartilage. In humans, the total body content of calcium is present mostly in the form of bone mineral (roughly 99%). In this state, it is largely unavailable for exchange/bioavailability. The way to overcome this is through the process of bone resorption, in which calcium is liberated into the bloodstream through the action of bone osteoclasts. The remainder of calcium is present within the extracellular and intracellular fluids.
Within a typical cell, the intracellular concentration of ionized calcium is roughly 100 nM, but is subject to increases of 10– to 100-fold during various cellular functions. The intracellular calcium level is kept relatively low with respect to the extracellular fluid, by an approximate magnitude of 12,000-fold. This gradient is maintained through various plasma membrane calcium pumps that utilize ATP for energy, as well as a sizable storage within intracellular compartments. In electrically excitable cells, such as skeletal and cardiac muscles and neurons, membrane depolarization leads to a Ca2+ transient with cytosolic Ca2+ concentration reaching 400 nM and above. Mitochondria are capable of sequestering and storing some of that Ca2+. It has been estimated that mitochondrial matrix free calcium concentration rises to the tens of micromolar levels in situ during neuronal activity.[6]
Effects
The effects of calcium on human cells are specific, meaning that different types of cells respond in different ways. However, in certain circumstances, its action may be more general. Ca2+ ions are one of the most widespread second messengers used in signal transduction. They make their entrance into the cytoplasm either from outside the cell through the cell membrane via calcium channels (such as Calcium-binding proteins or voltage-gated calcium channels), or from some internal calcium storages such as the endoplasmic reticulum[3] and mitochondria. Levels of intracellular calcium are regulated by transport proteins that remove it from the cell. For example, the sodium-calcium exchanger uses energy from the electrochemical gradient of sodium by coupling the influx of sodium into cell (and down its concentration gradient) with the transport of calcium out of the cell. In addition, the plasma membrane Ca2+ ATPase (PMCA) obtains energy to pump calcium out of the cell by hydrolysing adenosine triphosphate (ATP). In neurons, voltage-dependent, calcium-selective ion channels are important for synaptic transmission through the release of neurotransmitters into the synaptic cleft by vesicle fusion of synaptic vesicles.
Calcium's function in muscle contraction was found as early as 1882 by Ringer. Subsequent investigations were to reveal its role as a messenger about a century later. Because its action is interconnected with cAMP, they are called synarchic messengers. Calcium can bind to several different calcium-modulated proteins such as troponin-C (the first one to be identified) and calmodulin, proteins that are necessary for promoting contraction in muscle.
In the endothelial cells which line the inside of blood vessels, Ca2+ ions can regulate several signaling pathways which cause the smooth muscle surrounding blood vessels to relax. Some of these Ca2+-activated pathways include the stimulation of eNOS to produce nitric oxide, as well as the stimulation of Kca channels to efflux K+ and cause hyperpolarization of the cell membrane. Both nitric oxide and hyperpolarization cause the smooth muscle to relax in order to regulate the amount of tone in blood vessels.[7] However, dysfunction within these Ca2+-activated pathways can lead to an increase in tone caused by unregulated smooth muscle contraction. This type of dysfunction can be seen in cardiovascular diseases, hypertension, and diabetes.[8]
Cell type | Effect |
---|---|
endothelial cells | ↑vasodilation |
secretory cells (mostly) | ↑secretion (vesicle fusion) |
juxtaglomerular cell | ↓secretion[9] |
Parathyroid chief cells | ↓secretion[9] |
Neurons | transmission (vesicle fusion) |
T cells | Activation in response to antigen presentation to the T cell receptor[10] |
myocytes |
|
Various | Activation of protein kinase C Further reading: Function of protein kinase C |

Negative effects and pathology
Substantial decreases in extracellular Ca2+ ion concentrations may result in a condition known as hypocalcemic tetany, which is marked by spontaneous motor neuron discharge. In addition, severe hypocalcaemia will begin to affect aspects of blood coagulation and signal transduction.
Ca2+ ions can damage cells if they enter in excessive numbers (for example, in the case of excitotoxicity, or over-excitation of neural circuits, which can occur in neurodegenerative diseases, or after insults such as brain trauma or stroke). Excessive entry of calcium into a cell may damage it or even cause it to undergo apoptosis, or death by necrosis. Calcium also acts as one of the primary regulators of osmotic stress (Osmotic shock). Chronically elevated plasma calcium (hypercalcemia) is associated with cardiac arrhythmias and decreased neuromuscular excitability. One cause of hypercalcemia is a condition known as hyperparathyroidism.
Invertebrates
Some invertebrates use calcium compounds for building their exoskeleton (shells and carapaces) or endoskeleton (echinoderm plates and poriferan calcareous spicules).
Plants
Stomata closing
When ABA signals the guard cells, free Ca2+ ions enter the cytosol from both outside the cell and internal stores, reversing the concentration gradient so the K+ ions begin exiting the cell. The loss of solutes makes the cell flaccid and closes the stomatal pores.
Cellular division
Calcium is a necessary ion in the formation of the mitotic spindle. Without the mitotic spindle, cellular division cannot occur. Although young leaves have a higher need for calcium, older leaves contain higher amounts of calcium because calcium is relatively immobile through the plant. It is not transported through the phloem because it can bind with other nutrient ions and precipitate out of liquid solutions.
Structural roles
Ca2+ ions are an essential component of plant cell walls and cell membranes, and are used as cations to balance organic anions in the plant vacuole.[11] The Ca2+ concentration of the vacuole may reach millimolar levels. The most striking use of Ca2+ ions as a structural element in algae occurs in the marine coccolithophores, which use Ca2+ to form the calcium carbonate plates, with which they are covered.
Calcium is needed to form the pectin in the middle lamella of newly formed cells.
Calcium is needed to stabilize the permeability of cell membranes. Without calcium, the cell walls are unable to stabilize and hold their contents. This is particularly important in developing fruits. Without calcium, the cell walls are weak and unable to hold the contents of the fruit.
Some plants accumulate Ca in their tissues, thus making them more firm. Calcium is stored as Ca-oxalate crystals in plastids.
Calcium coordination plays an important role in defining the structure and function of proteins. An example a protein with calcium coordination is von Willebrand factor (vWF) which has an essential role in blood clot formation process. It is discovered -using single molecule optical tweezers measurement - that calcium-bound vWF acts as a shear force sensor in the blood. Shear force leads to unfolding of the A2 domain of vWF whose refolding rate is dramatically enhanced in the presence of calcium.[12]
Cell signaling
Ca2+ ions are usually kept at nanomolar levels in the cytosol of plant cells, and act in a number of signal transduction pathways as second messengers.
Protists
Many protists make use of calcium.
Measurement
The amount of calcium in blood (more specifically, in blood plasma) can be measured as total calcium, which includes both protein-bound and free calcium. In contrast, ionized calcium is a measure of free calcium. An abnormally high level of calcium in plasma is termed hypercalcemia and an abnormally low level is termed hypocalcemia, with "abnormal" generally referring to levels outside the reference range.
Target | Lower limit | Upper limit | Unit |
Ionized calcium | 1.03,[13] 1.10[14] | 1.23,[13] 1.30[14] | mmol/L |
4.1,[15] 4.4[15] | 4.9,[15] 5.2[15] | mg/dL | |
Total calcium | 2.1,[16][17] 2.2[14] | 2.5,[14][17] 2.6,[17] 2.8[16] | mmol/L |
8.4,[16] 8.5[18] | 10.2,[16] 10.5[18] | mg/dL |
The main methods to measure serum calcium are:[19]
- O-Cresolphalein Complexone Method; A disadvantage of this method is that the volatile nature of the 2-Amino-2-Methyl-1-Propanol used in this method makes it necessary to calibrate the method every few hours in a clinical laboratory setup.
- Arsenazo III Method; This method is more robust, but the arsenic in the reagent is a health hazard.
The total amount of Ca2+ present in a tissue may be measured using Atomic absorption spectroscopy, in which the tissue is vaporized and combusted. To measure Ca2+ concentration or spatial distribution within the cell cytoplasm in vivo, a range of fluorescent reporters may be used. These include cell permeable, calcium-binding fluorescent dyes such as Fura-2 or genetically engineered variant of green fluorescent protein (GFP) named Cameleon.
Corrected calcium
As access to an ionized calcium is not always available a corrected calcium may be used instead. To calculate a corrected calcium in mmo/l on takes the total calcium in mmol/L and adds 0.02 multiple by (40 minus the serum albumin in g/L).[20]
Food sources
The USDA web site has a very complete table of calcium content (in mg) of common foods per common measures (link below).
Calcium amount in foods, 100 g:
- parmesan (cheese) = 1140 mg
- milk powder = 909 mg
- goat hard cheese = 895 mg
- Cheddar cheese = 720 mg
- tahini paste = 427 mg
- molasses = 273 mg
- almonds = 234 mg
- goat milk = 134 mg
- sesame seeds (unhulled) = 125 mg
- nonfat cow milk = 122 mg
- plain whole-milk yogurt = 121 mg
- hazelnuts = 114 mg
- ricotta (skimmed milk cheese) = 90 mg
- brown sugar = 85 mg
- lentils = 79 mg
- wheat germs = 72 mg
- pigeon peas = 62.7 mg
- chickpeas = 53.1
- eggs, boiled = 50 mg
- flour = 41 mg
- orange = 40 mg
- human milk = 33 mg
- rice, white, long-grain, parboiled, enriched, cooked = 19 mg
- trout = 19 mg
- beef = 12 mg
- cod = 11 mg
- horse meat = 10 mg
- honey = 5 mg
- white sugar = 0 mg
See also
References
- 1 2 Brini, Marisa; Ottolini, Denis; Calì, Tito; Carafoli, Ernesto (2013). "Chapter 4. Calcium in Health and Disease". In Astrid Sigel, Helmut Sigel and Roland K. O. Sigel. Interrelations between Essential Metal Ions and Human Diseases. Metal Ions in Life Sciences. 13. Springer. pp. 81–137. doi:10.1007/978-94-007-7500-8_4.
- 1 2 Brini, Marisa; Call, Tito; Ottolini, Denis; Carafoli, Ernesto (2013). "Chapter 5 Intracellular Calcium Homeostasis and Signaling". In Banci, Lucia (Ed.). Metallomics and the Cell. Metal Ions in Life Sciences. 12. Springer. doi:10.1007/978-94-007-5561-1_5. ISBN 978-94-007-5560-4. electronic-book ISBN 978-94-007-5561-1 ISSN 1559-0836 electronic-ISSN 1868-0402
- 1 2 Wilson, C.H.; Ali, E.S.; Scrimgeour, N.; Martin, A.M.; Hua, J.; Tallis, G.A.; Rychkov, G.Y.; Barritt, G.J. (2015). "Steatosis inhibits liver cell store-operated Ca(2)(+) entry and reduces ER Ca(2)(+) through a protein kinase C-dependent mechanism". Biochem J. 466: 379–390. doi:10.1042/bj20140881.
- ↑ Brini, Marisa; Ottolini, Denis; Calì, Tito; Carafoli, Ernesto (2013). "Chapter 4. Calcium in Health and Disease". In Astrid Sigel, Helmut Sigel and Roland K. O. Sigel. Interrelations between Essential Metal Ions and Human Diseases. Metal Ions in Life Sciences. 13. Springer. pp. 81–138. doi:10.1007/978-94-007-7500-8_4.
- ↑ Boron, Walter F.; Boulpaep, Emile L (2003). "The Parathyroid Glands and Vitamin D". Medical Physiology: A Cellular And Molecular Approach. Elsevier/Saunders. p. 1094. ISBN 1-4160-2328-3.
- ↑ Ivannikov, M.; et al. (2013). "Mitochondrial Free Ca2+ Levels and Their Effects on Energy Metabolism in Drosophila Motor Nerve Terminals". Biophys. J. 104 (11): 2353–2361. doi:10.1016/j.bpj.2013.03.064. PMC 3672877
. PMID 23746507.
- ↑ Christopher J Garland, C Robin Hiley, Kim A Dora. EDHF: spreading the influence of the endothelium. British Journal of Pharmacology. 164:3, 839-852. (2011).
- ↑ Hua Cai, David G. Harrison. Endothelial Dysfunction in Cardiovascular Diseases: The Role of Oxidant Stress. Circulation Research. 87, 840-844. (2000).
- 1 2 Boron, Walter F.; Boulpaep, Emile L (2003). Medical Physiology: A Cellular And Molecular Approach. Elsevier/Saunders. p. 867. ISBN 1-4160-2328-3.
- ↑ Levinson, Warren (2008). Review of medical microbiology and immunology. McGraw-Hill Medical. p. 414. ISBN 0-07-149620-3.
- ↑ White, Philip J.; Martin R. Broadley (2003). "Calcium in Plants". Annals of Botany. 92 (4): 487–511. doi:10.1093/aob/mcg164. PMID 12933363.
- ↑ Jakobi AJ, Mashaghi A, Tans SJ, Huizinga EG. Calcium modulates force sensing by the von Willebrand factor A2 domain. Nature Commun. 2011 Jul 12;2:385.
- 1 2 Larsson L, Ohman S (November 1978). "Serum ionized calcium and corrected total calcium in borderline hyperparathyroidism". Clin. Chem. 24 (11): 1962–5. PMID 709830.
- 1 2 3 4 Reference range list from Uppsala University Hospital ("Laborationslista"). Artnr 40284 Sj74a. Issued on April 22, 2008
- 1 2 3 4 Derived from molar values using molar mass of 40.08 g•mol−1
- 1 2 3 4 Last page of Deepak A. Rao; Le, Tao; Bhushan, Vikas (2007). First Aid for the USMLE Step 1 2008 (First Aid for the Usmle Step 1). McGraw-Hill Medical. ISBN 0-07-149868-0.
- 1 2 3 Derived from mass values using molar mass of 40.08 g•mol−1
- 1 2 Blood Test Results - Normal Ranges Bloodbook.Com
- ↑ Clin Chem. 1992 Jun;38(6):904-8. Single stable reagent (Arsenazo III) for optically robust measurement of calcium in serum and plasma. Leary NO, Pembroke A, Duggan PF.
- ↑ Minisola, S; Pepe, J; Piemonte, S; Cipriani, C (2 June 2015). "The diagnosis and management of hypercalcaemia.". BMJ (Clinical research ed.). 350: h2723. PMID 26037642.