Ocean acidification
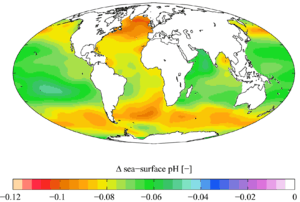
2 between the 1700s and the 1990s, from the Global Ocean Data Analysis Project (GLODAP) and the World Ocean Atlas
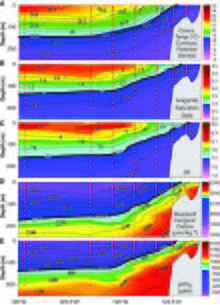
Ocean acidification is the ongoing decrease in the pH of the Earth's oceans, caused by the uptake of carbon dioxide (CO2) from the atmosphere.[2] Seawater is slightly basic (meaning pH > 7), and the process in question is a shift towards pH-neutral conditions rather than a transition to acidic conditions (pH < 7).[3] Ocean alkalinity is not changed by the process, or may increase over long time periods due to carbonate dissolution.[4] An estimated 30–40% of the carbon dioxide from human activity released into the atmosphere dissolves into oceans, rivers and lakes.[5][6] To achieve chemical equilibrium, some of it reacts with the water to form carbonic acid. Some of these extra carbonic acid molecules react with a water molecule to give a bicarbonate ion and a hydronium ion, thus increasing ocean acidity (H+ ion concentration). Between 1751 and 1996 surface ocean pH is estimated to have decreased from approximately 8.25 to 8.14,[7] representing an increase of almost 35% in H+ ion concentration in the world's oceans.[8][9] Earth System Models project that within the last decade ocean acidity exceeded historical analogs[10] and in combination with other ocean biogeochemical changes could undermine the functioning of marine ecosystems and disrupt the provision of many goods and services associated with the ocean.[11]
Increasing acidity is thought to have a range of potentially harmful consequences for marine organisms, such as depressing metabolic rates and immune responses in some organisms, and causing coral bleaching. By increasing the presence of free hydrogen ions, each molecule of carbonic acid that forms in the oceans ultimately results in the conversion of two carbonate ions into bicarbonate ions. This net decrease in the amount of carbonate ions available makes it more difficult for marine calcifying organisms, such as coral and some plankton, to form biogenic calcium carbonate, and such structures become vulnerable to dissolution.[12] Ongoing acidification of the oceans threatens food chains connected with the oceans.[13][14] As members of the InterAcademy Panel, 105 science academies have issued a statement on ocean acidification recommending that by 2050, global CO2 emissions be reduced by at least 50% compared to the 1990 level.[15]
While ongoing ocean acidification is anthropogenic in origin, it has occurred previously in Earth's history.[16] The most notable example is the Paleocene-Eocene Thermal Maximum (PETM),[17] which occurred approximately 56 million years ago. For reasons that are currently uncertain, massive amounts of carbon entered the ocean and atmosphere, and led to the dissolution of carbonate sediments in all ocean basins.
Ocean acidification has been called the "evil twin of global warming"[18][19][20][21][22] and "the other CO2 problem".[19][21][23]
Carbon cycle

2 cycle between the atmosphere and the ocean
The carbon cycle describes the fluxes of carbon dioxide (CO
2) between the oceans, terrestrial biosphere, lithosphere,[24] and the atmosphere. Human activities such as the combustion of fossil fuels and land use changes have led to a new flux of CO
2 into the atmosphere. About 45% has remained in the atmosphere; most of the rest has been taken up by the oceans,[25] with some taken up by terrestrial plants.[26]
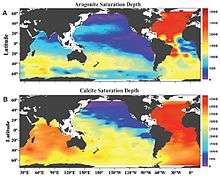
.png)
The carbon cycle involves both organic compounds such as cellulose and inorganic carbon compounds such as carbon dioxide and the carbonates. The inorganic compounds are particularly relevant when discussing ocean acidification for it includes many forms of dissolved CO
2 present in the Earth's oceans.[28]
When CO
2 dissolves, it reacts with water to form a balance of ionic and non-ionic chemical species: dissolved free carbon dioxide (CO
2(aq)), carbonic acid (H
2CO
3), bicarbonate (HCO−
3) and carbonate (CO2−
3). The ratio of these species depends on factors such as seawater temperature and alkalinity (as shown in a Bjerrum plot). These different forms of dissolved inorganic carbon are transferred from an ocean's surface to its interior by the ocean's solubility pump.
The resistance of an area of ocean to absorbing atmospheric CO
2 is known as the Revelle factor.
Acidification
Dissolving CO
2 in seawater increases the hydrogen ion (H+
) concentration in the ocean, and thus decreases ocean pH, as follows:[29]
Caldeira and Wickett (2003)[2] placed the rate and magnitude of modern ocean acidification changes in the context of probable historical changes during the last 300 million years.
Since the industrial revolution began, it is estimated that surface ocean pH has dropped by slightly more than 0.1 units on the logarithmic scale of pH, representing about a 29% increase in H+
. It is expected to drop by a further 0.3 to 0.5 pH units[11] (an additional doubling to tripling of today's post-industrial acid concentrations) by 2100 as the oceans absorb more anthropogenic CO
2, the impacts being most severe for coral reefs and the Southern Ocean.[2][12][30] These changes are predicted to continue rapidly as the oceans take up more anthropogenic CO
2 from the atmosphere. The degree of change to ocean chemistry, including ocean pH, will depend on the mitigation and emissions pathways[31] society takes.[32]
Although the largest changes are expected in the future,[12] a report from NOAA scientists found large quantities of water undersaturated in aragonite are already upwelling close to the Pacific continental shelf area of North America.[33] Continental shelves play an important role in marine ecosystems since most marine organisms live or are spawned there, and though the study only dealt with the area from Vancouver to Northern California, the authors suggest that other shelf areas may be experiencing similar effects.[33]
Time | pH | pH change relative to pre-industrial |
Source | H+ concentration change relative to pre-industrial |
---|---|---|---|---|
Pre-industrial (18th century) | 8.179 | analysed field[34] | ||
Recent past (1990s) | 8.104 | −0.075 | field[34] | + 18.9% |
Present levels | ~8.069 | −0.11 | field[8][9][35][36] | + 28.8% |
2050 (2×CO 2 = 560 ppm) |
7.949 | −0.230 | model[12] | + 69.8% |
2100 (IS92a)[37] | 7.824 | −0.355 | model[12] | + 126.5% |
Rate
“ | If we continue emitting CO2 at the same rate, by 2100 ocean acidity will increase by about 150 percent, a rate that has not been experienced for at least 400,000 years. | ” |
— UK Ocean Acidification Research Programme, 2015[38] |
One of the first detailed datasets to examine how pH varied over a period of time at a temperate coastal location found that acidification was occurring much faster than previously predicted, with consequences for near-shore benthic ecosystems.[39][40] Thomas Lovejoy, former chief biodiversity advisor to the World Bank, has suggested that "the acidity of the oceans will more than double in the next 40 years. This rate is 100 times faster than any changes in ocean acidity in the last 20 million years, making it unlikely that marine life can somehow adapt to the changes."[41] It is predicted that, by the year 2100, the level of acidity in the ocean will reach the levels experienced by the earth 20 million years ago.[11][42]
Current rates of ocean acidification have been compared with the greenhouse event at the Paleocene–Eocene boundary (about 55 million years ago) when surface ocean temperatures rose by 5–6 degrees Celsius. No catastrophe was seen in surface ecosystems, yet bottom-dwelling organisms in the deep ocean experienced a major extinction. The current acidification is on a path to reach levels higher than any seen in the last 65 million years,[43] and the rate of increase is about ten times the rate that preceded the Paleocene–Eocene mass extinction. The current and projected acidification has been described as an almost unprecedented geological event.[44] A National Research Council study released in April 2010 likewise concluded that "the level of acid in the oceans is increasing at an unprecedented rate."[45][46] A 2012 paper in the journal Science examined the geological record in an attempt to find a historical analog for current global conditions as well as those of the future. The researchers determined that the current rate of ocean acidification is faster than at any time in the past 300 million years.[47][48]
A review by climate scientists at the RealClimate blog, of a 2005 report by the Royal Society of the UK similarly highlighted the centrality of the rates of change in the present anthropogenic acidification process, writing:[49]
"The natural pH of the ocean is determined by a need to balance the deposition and burial of CaCO
3 on the sea floor against the influx of Ca2+
and CO2−
3 into the ocean from dissolving rocks on land, called weathering. These processes stabilize the pH of the ocean, by a mechanism called CaCO
3 compensation...The point of bringing it up again is to note that if the CO
2 concentration of the atmosphere changes more slowly than this, as it always has throughout the Vostok record, the pH of the ocean will be relatively unaffected because CaCO
3 compensation can keep up. The [present] fossil fuel acidification is much faster than natural changes, and so the acid spike will be more intense than the earth has seen in at least 800,000 years."
In the 15-year period 1995–2010 alone, acidity has increased 6 percent in the upper 100 meters of the Pacific Ocean from Hawaii to Alaska.[50] According to a statement in July 2012 by Jane Lubchenco, head of the U.S. National Oceanic and Atmospheric Administration "surface waters are changing much more rapidly than initial calculations have suggested. It's yet another reason to be very seriously concerned about the amount of carbon dioxide that is in the atmosphere now and the additional amount we continue to put out."[18]
A 2013 study claimed acidity was increasing at a rate 10 times faster than in any of the evolutionary crises in Earth's history.[51] In a synthesis report published in Science in 2015, 22 leading marine scientists stated that CO2 from burning fossil fuels is changing the oceans' chemistry more rapidly than at any time since the Great Dying, Earth's most severe known extinction event, emphasizing that the 2 °C maximum temperature increase agreed upon by governments reflects too small a cut in emissions to prevent "dramatic impacts" on the world's oceans, with lead author Jean-Pierre Gattuso remarking that "The ocean has been minimally considered at previous climate negotiations. Our study provides compelling arguments for a radical change at the UN conference (in Paris) on climate change".[52]
Calcification
Overview
Changes in ocean chemistry can have extensive direct and indirect effects on organisms and their habitats. One of the most important repercussions of increasing ocean acidity relates to the production of shells and plates out of calcium carbonate (CaCO
3).[30] This process is called calcification and is important to the biology and survival of a wide range of marine organisms. Calcification involves the precipitation of dissolved ions into solid CaCO
3 structures, such as coccoliths. After they are formed, such structures are vulnerable to dissolution unless the surrounding seawater contains saturating concentrations of carbonate ions (CO32−).
Mechanism
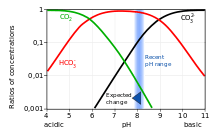
Of the extra carbon dioxide added into the oceans, some remains as dissolved carbon dioxide, while the rest contributes towards making additional bicarbonate (and additional carbonic acid). This also increases the concentration of hydrogen ions, and the percentage increase in hydrogen is larger than the percentage increase in bicarbonate,[53] creating an imbalance in the reaction HCO3− CO32− + H+. To maintain chemical equilibrium, some of the carbonate ions already in the ocean combine with some of the hydrogen ions to make further bicarbonate. Thus the ocean's concentration of carbonate ions is reduced, creating an imbalance in the reaction Ca2+ + CO32− CaCO3, and making the dissolution of formed CaCO
3 structures more likely.
These increases in concentrations of dissolved carbon dioxide and bicarbonate, and reduction in carbonate, are shown in a Bjerrum plot.
Saturation state
The saturation state (known as Ω) of seawater for a mineral is a measure of the thermodynamic potential for the mineral to form or to dissolve, and is described by the following equation:
Here Ω is the product of the concentrations (or activities) of the reacting ions that form the mineral (Ca2+
and CO2−
3), divided by the product of the concentrations of those ions when the mineral is at equilibrium (K
sp), that is, when the mineral is neither forming nor dissolving.[54] In seawater, a natural horizontal boundary is formed as a result of temperature, pressure, and depth, and is known as the saturation horizon, or lysocline.[30] Above this saturation horizon, Ω has a value greater than 1, and CaCO
3 does not readily dissolve. Most calcifying organisms live in such waters.[30] Below this depth, Ω has a value less than 1, and CaCO
3 will dissolve. However, if its production rate is high enough to offset dissolution, CaCO
3 can still occur where Ω is less than 1. The carbonate compensation depth occurs at the depth in the ocean where production is exceeded by dissolution.[55]
The decrease in the concentration of CO32− decreases Ω, and hence makes CaCO
3 dissolution more likely.
Calcium carbonate occurs in two common polymorphs (crystalline forms): aragonite and calcite. Aragonite is much more soluble than calcite, so the aragonite saturation horizon is always nearer to the surface than the calcite saturation horizon.[30] This also means that those organisms that produce aragonite may be more vulnerable to changes in ocean acidity than those that produce calcite.[12] Increasing CO
2 levels and the resulting lower pH of seawater decreases the saturation state of CaCO
3 and raises the saturation horizons of both forms closer to the surface.[56] This decrease in saturation state is believed to be one of the main factors leading to decreased calcification in marine organisms, as the inorganic precipitation of CaCO
3 is directly proportional to its saturation state.[57]
Possible impacts
.webm.jpg)
Increasing acidity has possibly harmful consequences, such as depressing metabolic rates in jumbo squid,[58] depressing the immune responses of blue mussels,[59] and coral bleaching. However it may benefit some species, for example increasing the growth rate of the sea star, Pisaster ochraceus,[60] while shelled plankton species may flourish in altered oceans.[61]
The report "Ocean Acidification Summary for Policymakers 2013" describes research findings and possible impacts.[62]
Impacts on oceanic calcifying organisms
Although the natural absorption of CO
2 by the world's oceans helps mitigate the climatic effects of anthropogenic emissions of CO
2, it is believed that the resulting decrease in pH will have negative consequences, primarily for oceanic calcifying organisms. These span the food chain from autotrophs to heterotrophs and include organisms such as coccolithophores, corals, foraminifera, echinoderms, crustaceans and molluscs.[11][63] As described above, under normal conditions, calcite and aragonite are stable in surface waters since the carbonate ion is at supersaturating concentrations. However, as ocean pH falls, the concentration of carbonate ions required for saturation to occur increases, and when carbonate becomes undersaturated, structures made of calcium carbonate are vulnerable to dissolution. Therefore, even if there is no change in the rate of calcification, the rate of dissolution of calcareous material increases.[64]
Corals,[65][66][67] coccolithophore algae,[68][69][70][71] coralline algae,[72] foraminifera,[73] shellfish[74] and pteropods[12][75] experience reduced calcification or enhanced dissolution when exposed to elevated CO
2.
The Royal Society published a comprehensive overview of ocean acidification, and its potential consequences, in June 2005.[30] However, some studies have found different response to ocean acidification, with coccolithophore calcification and photosynthesis both increasing under elevated atmospheric pCO2,[76][77][78] an equal decline in primary production and calcification in response to elevated CO2[79] or the direction of the response varying between species.[80] A study in 2008 examining a sediment core from the North Atlantic found that while the species composition of coccolithophorids has remained unchanged for the industrial period 1780 to 2004, the calcification of coccoliths has increased by up to 40% during the same time.[78] A 2010 study from Stony Brook University suggested that while some areas are overharvested and other fishing grounds are being restored, because of ocean acidification it may be impossible to bring back many previous shellfish populations.[81] While the full ecological consequences of these changes in calcification are still uncertain, it appears likely that many calcifying species will be adversely affected.
When exposed in experiments to pH reduced by 0.2 to 0.4, larvae of a temperate brittlestar, a relative of the common sea star, fewer than 0.1 percent survived more than eight days.[50] There is also a suggestion that a decline in the coccolithophores may have secondary effects on climate, contributing to global warming by decreasing the Earth's albedo via their effects on oceanic cloud cover.[82] All marine ecosystems on Earth will be exposed to changes in acidification and several other ocean biogeochemical changes.[11]
The fluid in the internal compartments where corals grow their exoskeleton is also extremely important for calcification growth. When the saturation rate of aragonite in the external seawater is at ambient levels, the corals will grow their aragonite crystals rapidly in their internal compartments, hence their exoskeleton grows rapidly. If the level of aragonite in the external seawater is lower than the ambient level, the corals have to work harder to maintain the right balance in the internal compartment. When that happens, the process of growing the crystals slows down, and this slows down the rate of how much their exoskeleton is growing. Depending on how much aragonite is in the surrounding water, the corals may even stop growing because the levels of aragonite are too low to pump in to the internal compartment. They could even dissolve faster than they can make the crystals to their skeleton, depending on the aragonite levels in the surrounding water.[83]
Ocean acidification may force some organisms to reallocate resources away from productive endpoints such as growth in order to maintain calcification.[84]
In some places carbon dioxide bubbles out from the sea floor, locally changing the pH and other aspects of the chemistry of the seawater. Studies of these carbon dioxide seeps have documented a variety of responses by different organisms.[8] Coral reef communities located near carbon dioxide seeps are of particular interest because of the sensitivity of some corals species to acidification. In Papua New Guinea, declining pH caused by carbon dioxide seeps is associated with declines in coral species diversity.[85] However, in Palau carbon dioxide seeps are not associated with reduced species diversity of corals, although bioerosion of coral skeletons is much higher at low pH sites.
Other biological impacts
Aside from the slowing and/or reversing of calcification, organisms may suffer other adverse effects, either indirectly through negative impacts on food resources,[30] or directly as reproductive or physiological effects. For example, the elevated oceanic levels of CO2 may produce CO
2-induced acidification of body fluids, known as hypercapnia. Also, increasing ocean acidity is believed to have a range of direct consequences. For example, increasing acidity has been observed to: reduce metabolic rates in jumbo squid;[58] depress the immune responses of blue mussels;[59] and make it harder for juvenile clownfish to tell apart the smells of non-predators and predators,[86] or hear the sounds of their predators.[87] This is possibly because ocean acidification may alter the acoustic properties of seawater, allowing sound to propagate further, and increasing ocean noise.[88] Calcium carbonate ions are very important when it comes to building organisms as they use calcium to build skeletons and shells. OA affects ocean species to a varying degree. Many plants do well with high CO2 levels, but organisms that calcify like clams, mussels, corals and sea urchins might not do so well because everything in the ocean is connected including the food web. Since OA is such a large event because the ocean takes up 71% of the earth's surface OA is being referred to as climate change because it will affect the whole planet if the ocean becomes acidic. This impacts all animals that use sound for echolocation or communication.[89] Atlantic longfin squid eggs took longer to hatch in acidified water, and the squid's statolith was smaller and malformed in animals placed in sea water with a lower pH. The lower PH was simulated with 20-30 times the normal amount of CO2.[90] However, as with calcification, as yet there is not a full understanding of these processes in marine organisms or ecosystems.[91]
Another possible effect would be an increase in red tide events, which could contribute to the accumulation of toxins (domoic acid, brevetoxin, saxitoxin) in small organisms such as anchovies and shellfish, in turn increasing occurrences of amnesic shellfish poisoning, neurotoxic shellfish poisoning and paralytic shellfish poisoning.[92]
Nonbiological impacts
Leaving aside direct biological effects, it is expected that ocean acidification in the future will lead to a significant decrease in the burial of carbonate sediments for several centuries, and even the dissolution of existing carbonate sediments.[93] This will cause an elevation of ocean alkalinity, leading to the enhancement of the ocean as a reservoir for CO2 with implications for climate change as more CO2 leaves the atmosphere for the ocean.[94]
Impact on human industry
The threat of acidification includes a decline in commercial fisheries and in the Arctic tourism industry and economy. Commercial fisheries are threatened because acidification harms calcifying organisms which form the base of the Arctic food webs.
Pteropods and brittle stars both form the base of the Arctic food webs and are both seriously damaged from acidification. Pteropods shells dissolve with increasing acidification and the brittle stars lose muscle mass when re-growing appendages.[95] For pteropods to create shells they require aragonite which is produced through carbonate ions and dissolved calcium. Pteropods are severely affected because increasing acidification levels have steadily decreased the amount of water supersaturated with carbonate which is needed for aragonite creation.[96] Arctic waters are changing so rapidly that they will become undersaturated with aragonite as early as 2016.[96] Additionally the brittle star's eggs die within a few days when exposed to expected conditions resulting from Arctic acidification.[97] Acidification threatens to destroy Arctic food webs from the base up. Arctic food webs are considered simple, meaning there are few steps in the food chain from small organisms to larger predators. For example, pteropods are “a key prey item of a number of higher predators - larger plankton, fish, seabirds, whales"[98] Both pteropods and sea stars serve as a substantial food source and their removal from the simple food web would pose a serious threat to the whole ecosystem. The effects on the calcifying organisms at the base of the food webs could potentially destroy fisheries. The value of fish caught from US commercial fisheries in 2007 was valued at $3.8 billion and of that 73% was derived from calcifiers and their direct predators.[99] Other organisms are directly harmed as a result of acidification. For example, decrease in the growth of marine calcifiers such as the American Lobster, Ocean Quahog, and scallops means there is less shellfish meat available for sale and consumption.[100] Red king crab fisheries are also at a serious threat because crabs are calcifiers and rely on carbonate ions for shell development. Baby red king crab when exposed to increased acidification levels experienced 100% mortality after 95 days.[101] In 2006 Red King Cab accounted for 23% of the total guideline harvest levels and a serious decline in red crab population would threaten the crab harvesting industry.[102] Several ocean goods and services are likely to be undermined by future ocean acidification potentially affecting the livelihoods of some 400 to 800 million people depending upon the emission scenario.[11]
Impact on indigenous peoples
Acidification could damage the Arctic tourism economy and affect the way of life of indigenous peoples. A major pillar of Arctic tourism is the sport fishing and hunting industry. The sport fishing industry is threatened by collapsing food webs which provide food for the prized fish. A decline in tourism lowers revenue input in the area, and threatens the economies that are increasingly dependent on tourism.[103] Acidification is not merely a threat but has significantly declined whole fish populations. For example, In Scandinavia studies conducted on acidic water revealed that 15% of species populations had disappeared and that many more populations were limited in numbers or declining.[104] The rapid decrease or disappearance of marine life could also affect the diet of Indigenous peoples.
Possible responses
Reducing CO2 emissions
Members of the InterAcademy Panel recommended that by 2050, global anthropogenic CO2 emissions be reduced less than 50% of the 1990 level.[15] The 2009[15] statement also called on world leaders to:
- Acknowledge that ocean acidification is a direct and real consequence of increasing atmospheric CO2 concentrations, is already having an effect at current concentrations, and is likely to cause grave harm to important marine ecosystems as CO2 concentrations reach 450 [parts-per-million (ppm)] and above;
- [...] Recognise that reducing the build up of CO2 in the atmosphere is the only practicable solution to mitigating ocean acidification;
- [...] Reinvigorate action to reduce stressors, such as overfishing and pollution, on marine ecosystems to increase resilience to ocean acidification.
Stabilizing atmospheric CO2 concentrations at 450 ppm would require near-term emissions reductions, with steeper reductions over time.[105]
The German Advisory Council on Global Change[106] stated:
In order to prevent disruption of the calcification of marine organisms and the resultant risk of fundamentally altering marine food webs, the following guard rail should be obeyed: the pH of near surface waters should not drop more than 0.2 units below the pre-industrial average value in any larger ocean region (nor in the global mean).
One policy target related to ocean acidity is the magnitude of future global warming. Parties to the United Nations Framework Convention on Climate Change (UNFCCC) adopted a target of limiting warming to below 2 °C, relative to the pre-industrial level.[107] Meeting this target would require substantial reductions in anthropogenic CO2 emissions.[108]
Limiting global warming to below 2 °C would imply a reduction in surface ocean pH of 0.16 from pre-industrial levels. This would represent a substantial decline in surface ocean pH.[109]
Climate engineering
Climate engineering (mitigating temperature or pH effects of emissions) has been proposed as a possible response to ocean acidification. The IAP (2009)[15] statement cautioned against climate engineering as a policy response:
Mitigation approaches such as adding chemicals to counter the effects of acidification are likely to be expensive, only partly effective and only at a very local scale, and may pose additional unanticipated risks to the marine environment. There has been very little research on the feasibility and impacts of these approaches. Substantial research is needed before these techniques could be applied.
Reports by the WGBU (2006),[106] the UK's Royal Society (2009),[110] and the US National Research Council (2011)[111] warned of the potential risks and difficulties associated with climate engineering.
Iron fertilization
Iron fertilization of the ocean could stimulate photosynthesis in phytoplankton (see Iron Hypothesis). The phytoplankton would convert the ocean's dissolved carbon dioxide into carbohydrate and oxygen gas, some of which would sink into the deeper ocean before oxidizing. More than a dozen open-sea experiments confirmed that adding iron to the ocean increases photosynthesis in phytoplankton by up to 30 times.[112] While this approach has been proposed as a potential solution to the ocean acidification problem, mitigation of surface ocean acidification might increase acidification in the less-inhabited deep ocean.[113]
A report by the UK's Royal Society (2009)[114] reviewed the approach for effectiveness, affordability, timeliness and safety. The rating for affordability was "medium", or "not expected to be very cost-effective." For the other three criteria, the ratings ranged from "low" to "very low" (i.e., not good). For example, in regards to safety, the report found a "[high] potential for undesirable ecological side effects," and that ocean fertilization "may increase anoxic regions of ocean ('dead zones')."[115]
Carbon negative fuels
Carbonic acid can be extracted from seawater as carbon dioxide for use in making synthetic fuel.[116][117] If the resulting flue exhaust gas was subject to carbon capture, then the process would be carbon negative over time, resulting in permanent extraction of inorganic carbon from seawater and the atmosphere with which seawater is in equilibrium. Based on the energy requirements, this process was estimated to cost about $50 per tonne of CO
2.[118]
Gallery
-
"Present day" (1990s) sea surface pH
-
Present day alkalinity
-
"Present day" (1990s) sea surface anthropogenic CO
2 -
Vertical inventory of "present day" (1990s) anthropogenic CO
2 -
Change in surface CO2−
3 ion from the 1700s to the 1990s -
Present day DIC
-
Pre-Industrial DIC
See also
- Biological pump
- Carbon dioxide sinks
- Effect of global warming on oceans
- Estuarine acidification
- Ocean deoxygenation
- Holocene extinction
References
- ↑ "Feely et al. - Evidence for upwelling of corrosive "acidified" water onto the Continental Shel". pmel.noaa.gov. Retrieved 2014-01-25.
- 1 2 3 Caldeira, K.; Wickett, M. E. (2003). "Anthropogenic carbon and ocean pH". Nature. 425 (6956): 365–365. Bibcode:2001AGUFMOS11C0385C. doi:10.1038/425365a. PMID 14508477.
- ↑ The ocean would not become acidic even if it were to absorb the CO2 produced from the combustion of all fossil fuel resources.
- ↑ Kump, L.R.; Bralower, T.J.; Ridgwell, A. (2009). "Ocean acidification in deep time". Oceanography. 22: 94–107. doi:10.5670/oceanog.2009.10. Retrieved 16 May 2016.
- ↑ Millero, Frank J. (1995). "Thermodynamics of the carbon dioxide system in the oceans". Geochimica et Cosmochimica Acta. 59 (4): 661–677. Bibcode:1995GeCoA..59..661M. doi:10.1016/0016-7037(94)00354-O.
- ↑ Feely, R. A.; et al. (July 2004). "Impact of Anthropogenic CO2 on the CaCO3 System in the Oceans". Science. 305 (5682): 362–366. Bibcode:2004Sci...305..362F. doi:10.1126/science.1097329. PMID 15256664.
- ↑ Jacobson, M. Z. (2005). "Studying ocean acidification with conservative, stable numerical schemes for nonequilibrium air-ocean exchange and ocean equilibrium chemistry". Journal of Geophysical Research: Atmospheres. 110: D07302. Bibcode:2005JGRD..11007302J. doi:10.1029/2004JD005220.
- 1 2 3 Hall-Spencer, J. M.; Rodolfo-Metalpa, R.; Martin, S.; et al. (July 2008). "Volcanic carbon dioxide vents show ecosystem effects of ocean acidification". Nature. 454 (7200): 96–9. Bibcode:2008Natur.454...96H. doi:10.1038/nature07051. PMID 18536730.
- 1 2 Report of the Ocean Acidification and Oxygen Working Group, International Council for Science's Scientific Committee on Ocean Research (SCOR) Biological Observatories Workshop
- ↑ Mora, C (2013). "The projected timing of climate departure from recent variability". Nature. 502: 183–187. doi:10.1038/nature12540.
- 1 2 3 4 5 6 Mora, C.; et al. (2013). "Biotic and Human Vulnerability to Projected Changes in Ocean Biogeochemistry over the 21st Century". PLoS Biology. 11: e1001682. doi:10.1371/journal.pbio.1001682. PMC 3797030
. PMID 24143135.
- 1 2 3 4 5 6 7 8 Orr, James C.; et al. (2005). "Anthropogenic ocean acidification over the twenty-first century and its impact on calcifying organisms" (PDF). Nature. 437 (7059): 681–686. Bibcode:2005Natur.437..681O. doi:10.1038/nature04095. PMID 16193043. Archived from the original (PDF) on 2008-06-25.
- ↑ Cornelia Dean (January 30, 2009). "Rising Acidity Is Threatening Food Web of Oceans, Science Panel Says". New York Times.
- ↑ Robert E. Service (13 July 2012). "Rising Acidity Brings and Ocean Of Trouble". Science. 337 (6091): 146–148. Bibcode:2012Sci...337..146S. doi:10.1126/science.337.6091.146. PMID 22798578.
- 1 2 3 4 IAP (June 2009). "Interacademy Panel (IAP) Member Academies Statement on Ocean Acidification"., Secretariat: TWAS (the Academy of Sciences for the Developing World), Trieste, Italy.
- ↑ Zeebe, R.E. (2012). "History of Seawater Carbonate Chemistry, Atmospheric CO2, and Ocean Acidification". Annual Review of Earth and Planetary Sciences. 40: 141–165. doi:10.1146/annurev-earth-042711-105521. Retrieved 16 May 2016.
- ↑ Zachos, J.C.; Röhl, U.; Schellenberg, S.A.; Sluijs, A.; Hodell, D.A.; Kelly, D.C.; Thomas, E.; Nicolo, M.; Raffi, I.; Lourens, L. J.; McCarren, H.; Kroon, D. (2005). "Rapid acidification of the ocean during the Paleocene-Eocene thermal maximum". Science. 308 (5728): 1611–1615. doi:10.1126/science.1109004. PMID 15947184.
- 1 2 Huffington Post, 9 July 2012, "Ocean Acidification Is Climate Change's 'Equally Evil Twin,' NOAA Chief Says," http://www.huffingtonpost.com/2012/07/09/ocean-acidification-reefs-climate-change_n_1658081.html?utm_hp_ref=green
- 1 2 The other carbon dioxide problem http://www.rsc.org/chemistryworld/2014/07/ocean-acidification
- ↑ Global warming’s evil twin: ocean acidification http://theconversation.com/global-warmings-evil-twin-ocean-acidification-19017
- 1 2 Hennige, S.J. (2014). "Short-term metabolic and growth responses of the cold-water coral Lophelia pertusa to ocean acidification". Deep-Sea Research Part II. 99: 27–35. doi:10.1016/j.dsr2.2013.07.005.
- ↑ Pelejero, C. (2010). "Paleo-perspectives on ocean acidification". Trends in Ecology and Evolution. 25 (6): 332–344. doi:10.1016/j.tree.2010.02.002.
- ↑ Doney, S.C. (2009). "Ocean Acidification: The Other CO2 Problem". Annual Review of Marine Science. 1: 169–192. Bibcode:2009ARMS....1..169D. doi:10.1146/annurev.marine.010908.163834.
- ↑ "carbon cycle". Encyclopædia Britannica Online. Retrieved 11 Feb 2010.
- ↑ Raven, J. A.; Falkowski, P. G. (1999). "Oceanic sinks for atmospheric CO2". Plant, Cell & Environment. 22 (6): 741–755. doi:10.1046/j.1365-3040.1999.00419.x.
- ↑ Cramer, W.; et al. (2001). "Global response of terrestrial ecosystem structure and function to CO2 and climate change: results from six dynamic global vegetation models". Global Change Biology. 7 (4): 357–373. doi:10.1046/j.1365-2486.2001.00383.x.
- ↑ "Feely et al. - Impact of Anthropogenic CO2 on the CaCO3 System in the Oceans". pmel.noaa.gov. Retrieved 2014-01-25.
- ↑ Kump, Lee R.; Kasting, James F.; Crane, Robert G. (2003). The Earth System (2nd ed.). Upper Saddle River: Prentice Hall. pp. 162–164. ISBN 0-613-91814-2.
- ↑ IPCC (2005). "IPCC Special Report on Carbon Dioxide Capture and Storage" (PDF): 390.
- 1 2 3 4 5 6 7 Raven, J. A. et al. (2005). Ocean acidification due to increasing atmospheric carbon dioxide. Royal Society, London, UK.
- ↑ Bows, Kevin; Bows, Alice (2011). "Beyond 'dangerous' climate change: emission scenarios for a new world" (PDF). Philosophical Transactions of the Royal Society A. 369 (1934): 20–44. Bibcode:2011RSPTA.369...20A. doi:10.1098/rsta.2010.0290. Retrieved 2011-05-22.
- ↑ Turley, C. (2008). "Impacts of changing ocean chemistry in a high-CO
2 world". Mineralogical Magazine. 72 (1): 359–362. doi:10.1180/minmag.2008.072.1.359. - 1 2 Feely, R. A.; Sabine, C. L.; Hernandez-Ayon, J. M.; Ianson, D.; Hales B. (June 2008). "Evidence for upwelling of corrosive "acidified" water onto the continental shelf". Science. 320 (5882): 1490–2. Bibcode:2008Sci...320.1490F. doi:10.1126/science.1155676. PMID 18497259.
- 1 2 Key, R. M.; Kozyr, A.; Sabine, C. L.; Lee, K.; Wanninkhof, R.; Bullister, J.; Feely, R. A.; Millero, F.; Mordy, C.; Peng, T.-H. (2004). "A global ocean carbon climatology: Results from GLODAP". Global Biogeochemical Cycles. 18 (4): GB4031. Bibcode:2004GBioC..18.4031K. doi:10.1029/2004GB002247.
- ↑ "Ocean acidification and the Southern Ocean" by the Australian Antarctic Division of the Australian Government
- ↑ EPA weighs action on ocean acidification post at official blog of EPOCA, the European Project on Ocean Acidification
- ↑ Review of Past IPCC Emissions Scenarios, IPCC Special Report on Emissions Scenarios (ISBN 0521804930).
- ↑ Cited in Tim Flannery, Atmosphere of Hope. Solutions to the Climate Crisis, Penguin Books, 2015, page 47 (ISBN 9780141981048).
- ↑ Wootton, J. T.; Pfister, C. A.; Forester, J. D. (2008). "Dynamic patterns and ecological impacts of declining ocean pH in a high-resolution multi-year dataset". Proceedings of the National Academy of Sciences. 105 (48): 18848–18853. Bibcode:2008PNAS..10518848W. doi:10.1073/pnas.0810079105. PMC 2596240
. PMID 19033205.
- ↑ "Ocean Growing More Acidic Faster Than Once Thought; Increasing Acidity Threatens Sea Life". Science Daily. 2008-11-26. Retrieved 26 November 2008.
- ↑ UN: Oceans are 30 percent more acidic than before fossil fuels
- ↑ "What is Ocean Acidification". NOAA. Retrieved 24 August 2013.
- ↑ "Rate of ocean acidification the fastest in 65 million years". Physorg.com. 2010-02-14. Retrieved 2013-08-29.
- ↑ "An Ominous Warning on the Effects of Ocean Acidification by Carl Zimmer: Yale Environment 360". e360.yale.edu. Retrieved 2014-01-25.
- ↑ Report: Ocean acidification rising at unprecedented rate
- ↑ United States National Research Council, 2010. Ocean Acidification: A National Strategy to Meet the Challenges of a Changing Ocean
- ↑ "The Geological Record of Ocean Acidification". JournalistsResource.org, retrieved 14 March 2012
- ↑ Hönisch, Bärbel; Ridgwell, Andy; Schmidt, Daniela N.; Thomas, E.; Gibbs, S. J.; Sluijs, A.; Zeebe, R.; Kump, L.; Martindale, R. C.; Greene, S. E.; Kiessling, W.; Ries, J.; Zachos, J. C.; Royer, D. L.; Barker, S.; Marchitto, T. M.; Moyer, R.; Pelejero, C.; Ziveri, P.; Foster, G. L.; Williams, B. (2012). "The Geological Record of Ocean Acidification". Science. 335 (6072): 1058–1063. Bibcode:2012Sci...335.1058H. doi:10.1126/science.1208277. PMID 22383840.
- ↑ The Acid Ocean – the Other Problem with CO2 Emission
- 1 2 How Acidification Threatens Oceans from the Inside Out
- ↑ Fiona Harvey, environment correspondent (2013-08-25). "Rising levels of acids in seas may endanger marine life, says study | Environment". The Guardian. Retrieved 2013-08-29.
- ↑ CO2 emissions threaten ocean crisis
- ↑ Mitchell, M. J.; et al. (2010). "A model of carbon dioxide dissolution and mineral carbonation kinetics". Proceedings of the Royal Society A. 466 (2117): 1265–1290. doi:10.1098/rspa.2009.0349.
- ↑ Atkinson, M.J.; Cuet, P. (2008). "Possible effects of ocean acidification on coral reef biogeochemistry: topics for research". Marine Ecology Progress Series. 373: 249–256. doi:10.3354/meps07867.
- ↑ Thurman, H.V.; Trujillo, A.P. (2004). Introductory Oceanography. Prentice Hall. ISBN 978-0-13-143888-0.
- ↑ The Royal Society. Ocean Acidification Due To Increasing Atmospheric Carbon Dioxide, The Clyvedon Press Ltd. (2005): 11.
- ↑ Marubini, F.; Ferrier-Pagès, C.; Furla, P.; Allemand, D. (2008). "Coral calcification responds to seawater acidification: a working hypothesis towards a physiological mechanism". Coral Reefs. 27 (3): 491–499. Bibcode:2008CorRe..27..491M. doi:10.1007/s00338-008-0375-6.
- 1 2 Rosa, R.; Seibel, B. (2008). "Synergistic effects of climate-related variables suggest future physiological impairment in a top oceanic predator". PNAS. 105 (52): 20776–20780. Bibcode:2008PNAS..10520776R. doi:10.1073/pnas.0806886105.
- 1 2 Bibby, R.; et al. (2008). "Effects of ocean acidification on the immune response of the blue mussel Mytilus edulis". Aquatic Biology. 2: 67–74. doi:10.3354/ab00037.
- ↑ Gooding, R.; et al. (2008). "Elevated water temperature and carbon dioxide concentration increase the growth of a keystone echinoderm". Proceedings of the National Academy of Sciences. 106: 9316–21. doi:10.1073/pnas.0811143106. PMC 2695056
. PMID 19470464.
- ↑ Some like it acidic April 17, 2013 Science News
- ↑ "Ocean Acidification Summary for Policymakers". IGBP.
- ↑ National Research Council. Overview of Climate Changes and Illustrative Impacts. Climate Stabilization Targets: Emissions, Concentrations, and Impacts over Decades to Millennia. Washington, DC: The National Academies Press, 2011. 1. Print.
- ↑ Nienhuis, S.; Palmer, A.; Harley, C. (2010). "Elevated CO2 affects shell dissolution rate but not calcification rate in a marine snail". Proceedings of the Royal Society B. 277 (1693): 2553–2558. doi:10.1098/rspb.2010.0206. PMC 2894921
. PMID 20392726.
- ↑ Gattuso, J.-P.; Frankignoulle, M.; Bourge, I.; Romaine, S.; Buddemeier, R. W. (1998). "Effect of calcium carbonate saturation of seawater on coral calcification". Global and Planetary Change. 18 (1–2): 37–46. Bibcode:1998GPC....18...37G. doi:10.1016/S0921-8181(98)00035-6.
- ↑ Gattuso, J.-P.; Allemand, D.; Frankignoulle, M. (1999). "Photosynthesis and calcification at cellular, organismal and community levels in coral reefs: a review on interactions and control by carbonate chemistry". American Zoologist. 39: 160–183. doi:10.1093/icb/39.1.160.
- ↑ Langdon, C.; Atkinson, M. J. (2005). "Effect of elevated pCO2 on photosynthesis and calcification of corals and interactions with seasonal change in temperature/irradiance and nutrient enrichment". Journal of Geophysical Research. 110 (C09S07): C09S07. Bibcode:2005JGRC..11009S07L. doi:10.1029/2004JC002576.
- ↑ Riebesell, Ulf; Zondervan, Ingrid; Rost, Björn; Tortell, Philippe D.; Zeebe, Richard E. and François M. M. Morel (2000). "Reduced calcification of marine plankton in response to increased atmospheric CO
2". Nature. 407 (6802): 364–367. doi:10.1038/35030078. PMID 11014189. - ↑ Zondervan, I.; Zeebe, R. E.; Rost, B.; Rieblesell, U. (2001). "Decreasing marine biogenic calcification: a negative feedback on rising atmospheric CO2". Global Biogeochemical Cycles. 15 (2): 507–516. Bibcode:2001GBioC..15..507Z. doi:10.1029/2000GB001321.
- ↑ Zondervan, I.; =Rost, B.; Rieblesell, U. (2002). "Effect of CO2 concentration on the PIC/POC ratio in the coccolithophore Emiliania huxleyi grown under light limiting conditions and different day lengths". Journal of Experimental Marine Biology and Ecology. 272 (1): 55–70. doi:10.1016/S0022-0981(02)00037-0.
- ↑ Delille, B.; Harlay, J.; Zondervan, I.; Jacquet, S.; Chou, L.; Wollast, R.; Bellerby, R.G.J.; Frankignoulle, M.; Borges, A.V.; Riebesell, U.; Gattuso, J.-P. (2005). "Response of primary production and calcification to changes of pCO2 during experimental blooms of the coccolithophorid Emiliania huxleyi". Global Biogeochemical Cycles. 19 (2): GB2023. Bibcode:2005GBioC..19.2023D. doi:10.1029/2004GB002318.
- ↑ Kuffner, I. B.; Andersson, A. J.; Jokiel, P. L.; Rodgers, K. S.; Mackenzie, F. T. (2007). "Decreased abundance of crustose coralline algae due to ocean acidification". Nature Geoscience. 1 (2): 114–117. Bibcode:2008NatGe...1..114K. doi:10.1038/ngeo100.
- ↑ Phillips, Graham; Chris Branagan (2007-09-13). "Ocean Acidification – The BIG global warming story". ABC TV Science: Catalyst. Australian Broadcasting Corporation. Retrieved 2007-09-18.
- ↑ Gazeau, F.; Quiblier, C.; Jansen, J. M.; Gattuso, J.-P.; Middelburg, J. J. and Heip, C. H. R. (2007). "Impact of elevated CO
2 on shellfish calcification". Geophysical Research Letters. 34 (7): L07603. Bibcode:2007GeoRL..3407603G. doi:10.1029/2006GL028554. - ↑ Comeau, C.; Gorsky, G.; Jeffree, R.; Teyssié, J.-L.; Gattuso, J.-P. (2009). "Impact of ocean acidification on a key Arctic pelagic mollusc ("Limacina helicina")". Biogeosciences. 6 (9): 1877–1882. doi:10.5194/bg-6-1877-2009.
- ↑ Buitenhuis, E. T.; de Baar, H. J. W.; Veldhuis, M. J. W. (1999). "Photosynthesis and calcification by Emiliania huxleyi (Prymnesiophyceae) as a function of inorganic carbon species". Journal of Phycology. 35 (5): 949–959. doi:10.1046/j.1529-8817.1999.3550949.x.
- ↑ Nimer, N. A.; =Merrett, M. J. (1993). "Calcification rate in Emiliania huxleyi Lohmann in response to light, nitrate and availability of inorganic carbon". New Phytologist. 123 (4): 673–677. doi:10.1111/j.1469-8137.1993.tb03776.x.
- 1 2 Iglesias-Rodriguez, M. D.; Halloran, P. R.; Rickaby, R. E. M.; Hall, I. R., Colmenero-Hidalgo, E.; Gittins, J.R.; Green, D.R.H., Tyrrell, T.; Gibbs, S.J.; von Dassow, P.; Rehm, E.; Armbrust, E.V.; Boessenkool, K.P. (2008). "Phytoplankton Calcification in a High-CO2 World". Science. 320 (5874): 336–340. Bibcode:2008Sci...320..336I. doi:10.1126/science.1154122. PMID 18420926.
- ↑ Sciandra, A.; Harlay, J.; Lefevre, D.; et al. (2003). "Response of coccolithophorid Emiliania huxleyi to elevated partial pressure of CO2 under nitrogen limitation". Marine Ecology Progress Series. 261: 111–112. doi:10.3354/meps261111.
- ↑ Langer, G.; Geisen, M.; Baumann, K. H.; et al. (2006). "Species-specific responses of calcifying algae to changing seawater carbonate chemistry". Geochemistry, Geophysics, Geosystems. 7 (9): Q09006. Bibcode:2006GGG.....709006L. doi:10.1029/2005GC001227.
- ↑ "Acidification Of Oceans May Contribute To Global Declines Of Shellfish, Study By Stony Brook Scientists Concludes" (Press release). School of Marine and Atmospheric Sciences at Stony Brook University. 27 September 2010. Retrieved 4 June 2012.
- ↑ Ruttiman, J. (2006). "Sick Seas". Nature. 442 (7106): 978–980. Bibcode:2006Natur.442..978R. doi:10.1038/442978a. PMID 16943816.
- ↑ Cohen, A.; Holcomb, M. (2009). "Why Corals Care About Ocean Acidification: Uncovering the Mechanism" (PDF). Oceanography. 24 (4): 118–127. doi:10.5670/oceanog.2009.102.
- ↑ Hannah L. Wood; John I. Spicer; Stephen Widdicombe (2008). "Ocean acidification may increase calcification rates, but at a cost". Proceedings of the Royal Society B. 275 (1644): 1767–1773. doi:10.1098/rspb.2008.0343. PMC 2587798
. PMID 18460426.
- ↑ Fabricius, Katharina (2011). "Losers and winners in coral reefs acclimatized to elevated carbon dioxide concentrations". Nature Climate Change. 1: 165–169. doi:10.1038/nclimate1122.
- ↑ Dixson, D. L.; et al. (2010). "Ocean acidification disrupts the innate ability of fish to detect predator olfactory cues". Ecology Letters. 13 (1): 68–75. doi:10.1111/j.1461-0248.2009.01400.x. PMID 19917053.
- ↑ Simpson, S. D.; et al. (2011). "Ocean acidification erodes crucial auditory behaviour in a marine fish". Biology Letters. 7 (6): 917–20. doi:10.1098/rsbl.2011.0293. PMC 3210647
. PMID 21632617.
- ↑ Hester, K. C.; et al. (2008). "Unanticipated consequences of ocean acidification: A noisier ocean at lower pH" (PDF). Geophysical Research Letters. 35 (19). Bibcode:2008GeoRL..3519601H. doi:10.1029/2008GL034913.
- ↑ Acid In The Oceans: A Growing Threat To Sea Life by Richard Harris. All Things Considered, 12 August 2009.
- ↑ Kwok, Roberta. "Ocean acidification could make squid develop abnormally". University of Washington. Retrieved 2013-08-24.
- ↑ "Swiss marine researcher moving in for the krill". The Australian. 2008.
- ↑ "Ocean Acidification Promotes Disruptive and Harmful Algal Blooms on Our Coasts". 2014.
- ↑ Ridgwell, A.; Zondervan, I.; Hargreaves, J. C.; Bijma, J.; Lenton, T. M. (2007). "Assessing the potential long-term increase of oceanic fossil fuel CO2 uptake due to CO2-calcification feedback". Biogeosciences. 4 (4): 481–492. doi:10.5194/bg-4-481-2007.
- ↑ Tyrrell, T. (2008). "Calcium carbonate cycling in future oceans and its influence on future climates". Journal of Plankton Research. 30 (2): 141–156. doi:10.1093/plankt/fbm105.
- ↑ "Effects of Ocean Acidification on Marine Species & Ecosystems". Report. OCEANA. Retrieved October 13, 2013.
- 1 2 Lischka S.; B ̈ udenbender J.; Boxhammer T.; Riebesell U. (15 April 2011). "Impact of ocean acidification and elevated temperatures on early juveniles of the polar shelled pteropod Limacina helicina : mortality, shell degradation, and shell growth" (PDF). Report. Biogeosciences. pp. 919–932. Retrieved 14 November 2013.
- ↑ "Comprehensive study of Arctic Ocean acidification". Study. CICERO. Retrieved 14 November 2013.
- ↑ "Antarctic marine wildlife is under threat, study finds". BBC Nature. Retrieved October 13, 2013.
- ↑ V. J. Fabry; C. Langdon; W. M. Balch; A. G. Dickson; R. A. Feely; B. Hales; D. A. Hutchins; J. A. Kleypas & C. L. Sabine. "Present and Future Impacts of Ocean Acidification on Marine Ecosystems and Biogeochemical Cycles" (PDF). Report of the Ocean Carbon and Biogeochemistry Scoping Workshop on Ocean Acidification Research.
- ↑ "Canada's State of the Oceans Report, 2012". Report. Fisheries and Oceans Canada. 2012. Retrieved 21 October 2013.
- ↑ Robert J. Foy; Mark Carls; Michael Dalton; Tom Hurst; W. Christopher Long; Dusanka Poljak; André E. Punt; Michael F. Sigler; Robert P. Stone; Katherine M. Swiney (Winter 2013). "CO 2 , pH, and Anticipating a Future under Ocean Acidification" (PDF). ONCORHYNCHUS. Vol. XXXIII no. 1. Retrieved 14 November 2013.
- ↑ "Bering Sea Crab Fishery". Report. Seafood Market Bulletin. November 2005. Retrieved 10 November 2013.
- ↑ Snyder, John. "Tourism in the Polar Regions: The Sustainability Challenge" (PDF). Report. UNEP, The International Ecotourism Society. Retrieved 13 October 2013.
- ↑ Muniz, I. P. (May 1984). "The Effects of Acidification on Scandinavian Freshwater Fish Fauna". Series B, Biological Sciences. 305 (1124): 517–528. Bibcode:1984RSPTB.305..517M. doi:10.1098/rstb.1984.0074. JSTOR 2396102.
- ↑ Table TS.2 (p.9) and Figure TS.10 (p.20), in: Technical Summary, in Clarke & others 2007
- 1 2 Halting ocean acidification in time, in: Summary for Policymakers, in WBGU 2006, p. 3
- ↑ UNFCCC. Conference of the Parties (COP) (15 March 2011). "Report of the Conference of the Parties on its sixteenth session, held in Cancun from 29 November to 10 December 2010. Addendum. Part two: Action taken by the Conference of the Parties at its sixteenth session" (PDF). Geneva, Switzerland: United Nations, p.3, paragraph 4. Documen available in UN languages and text format.
- ↑ Ch 2: Which emission pathways are consistent with a 2 °C or 1.5 °C temperature limit?, in UNEP 2010, pp. 28–29
- ↑ Good & others 2010, Executive Summary
- ↑ Summary, in UK Royal Society 2009, pp. ix-xii
- ↑ "Ch 5: Key Elements of America's Climate Choices". America's Climate Choices. Box 5.1: Geoengineering., in US NRC 2011, pp. 52–53
- ↑ Trujillo, Alan (2011). Essentials of Oceanography. Pearson Education, Inc. p. 157. ISBN 9780321668127.
- ↑ Cao, L.; Caldeira, K. (2010). "Can ocean iron fertilization mitigate ocean acidification?". Climatic Change. 99 (1–2): 303–311. doi:10.1007/s10584-010-9799-4.
- ↑ Sec 2.3.1 Ocean fertilisation methods, in Ch 2: Carbon dioxide removal techniques, in UK Royal Society 2009, pp. 16–19
- ↑ Table 2.8, in: Sec 2.3.1 Ocean fertilisation methods, in Ch 2: Carbon dioxide removal techniques, in UK Royal Society 2009, p. 18
- ↑ DiMascio, Felice; Willauer, Heather D.; Hardy, Dennis R.; Lewis, M. Kathleen; Williams, Frederick W. (July 23, 2010). Extraction of Carbon Dioxide from Seawater by an Electrochemical Acidification Cell. Part 1 - Initial Feasibility Studies (PDF) (memorandum report). Washington, DC: Chemistry Division, Navy Technology Center for Safety and Survivability, U.S. Naval Research Laboratory. Retrieved September 7, 2012.
- ↑ Willauer, Heather D.; DiMascio, Felice; Hardy, Dennis R.; Lewis, M. Kathleen; Williams, Frederick W. (April 11, 2011). Extraction of Carbon Dioxide from Seawater by an Electrochemical Acidification Cell. Part 2 - Laboratory Scaling Studies (PDF) (memorandum report). Washington, DC: Chemistry Division, Navy Technology Center for Safety and Survivability, U.S. Naval Research Laboratory. Retrieved September 7, 2012.
- ↑ Eisaman, Matthew D.; et al. (2012). "CO2 extraction from seawater using bipolar membrane electrodialysis" (PDF). Energy and Environmental Science. 5 (6): 7346–52. doi:10.1039/C2EE03393C. Retrieved September 7, 2012.
- Clarke, L.; et al. (July 2007). "Scenarios of Greenhouse Gas Emissions and Atmospheric Concentrations. Sub-report 2.1A of Synthesis and Assessment Product 2.1 by the U.S. Climate Change Science Program and the Subcommittee on Global Change Research". Washington, DC., USA: Department of Energy, Office of Biological & Environmental Research
- Good, P.; et al. (2010). "An updated review of developments in climate science research since IPCC AR4. A report by the AVOID consortium" (PDF). London, UK: Committee on Climate Change. Report website.
- UK Royal Society (September 2009). "Geoengineering the climate: science, governance and uncertainty" (PDF). London: UK Royal Society. ISBN 978-0-85403-773-5, RS Policy document 10/09. Report website.
- UNEP (November 2010). "The Emissions Gap Report: Are the Copenhagen Accord pledges sufficient to limit global warming to 2°C or 1.5°C? A preliminary assessment". Nairobi, Kenya: United Nations Environment Programme (UNEP). ISBN 978-92-807-3134-7
- US NRC (2011). America's Climate Choices. A report by the Committee on America's Climate Choices, US National Research Council (US NRC). Washington, DC, USA: National Academies Press. ISBN 978-0-309-14585-5
- WBGU (2006). Special Report: The Future Oceans – Warming Up, Rising High, Turning Sour (PDF). Berlin, Germany: WBGU. ISBN 3-936191-14-X. Report website.
Further reading
- Antarctic Climate and Ecosystems Cooperative Research Centre (ACE CRC) (2008). Position analysis: CO2 emissions and climate change: Ocean impacts and adaptation issues. ISSN 1835-7911. Hobart, Tasmania.
- Cicerone, R.; J. Orr; P. Brewer; et al. (2004). "The Ocean in a High CO
2 World" (PDF). Eos, Transactions, American Geophysical Union. American Geophysical Union. 85 (37): 351–353. Bibcode:2004EOSTr..85R.351C. doi:10.1029/2004EO370007. - Doney, S. C. (2006). "The Dangers of Ocean Acidification". Scientific American. 294 (3): 58–65. doi:10.1038/scientificamerican0306-58. ISSN 0036-8733. PMID 16502612., (Article preview only).
- Drake, J.L.; Mass, T.; Falkowski, P. G. (2014). "The evolution and future of carbonate precipitation in marine invertebrates: Witnessing extinction or documenting resilience in the Anthropocene?". Elementa. 2: 000026. doi:10.12952/journal.elementa.000026. ISSN 2325-1026.
- Feely, R. A.; Sabine, Christopher L.; Lee, Kitack; Berelson, Will; Kleypas, Joanie; Fabry, Victoria J.; Millero, Frank J. (2004). "Impact of Anthropogenic CO
2 on the CaCO
3 System in the Oceans". Science. 305 (5682): 362–366. Bibcode:2004Sci...305..362F. doi:10.1126/science.1097329. PMID 15256664. - Harrould-Kolieb, E.; Savitz, J. (2008). "Acid Test: Can We Save Our Oceans From CO2?". Oceana.
- Henderson, Caspar (2006-08-05). "Ocean acidification: the other CO2 problem". NewScientist.com news service. Archived from the original on 12 May 2008.
- Jacobson, M. Z. (2005). "Studying ocean acidification with conservative, stable numerical schemes for nonequilibrium air-ocean exchange and ocean equilibrium chemistry". Journal of Geophysical Research: Atmospheres. 110: D07302. Bibcode:2005JGRD..11007302J. doi:10.1029/2004JD005220.
- Kim, Rakhyun E. (2012). "Is a New Multilateral Environmental Agreement on Ocean Acidification Necessary?" (PDF). Review of European Community & International Environmental Law. 21 (3): 243–258. doi:10.1111/reel.12000.x.
- Kleypas, J. A., R. A. Feely, V. J. Fabry, C. Langdon, C. L. Sabine, and L. L. Robbins. (2006). Impacts of Ocean Acidification on Coral Reefs and Other Marine Calcifiers: A Guide for Further Research, report of a workshop held 18–20 April 2005, St. Petersburg, FL, sponsored by National Science Foundation, NOAA and the U.S. Geological Survey, 88pp.
- Kolbert, E. (2006-11-20). "The Darkening Sea: Carbon emissions and the ocean". The New Yorker.
- Mathis, J.T.; Feely, R. A. (2014). "Building an integrated coastal ocean acidification monitoring network in the U.S.". Elementa. 1: 000007. doi:10.12952/journal.elementa.000007. ISSN 2325-1026.
- Riebesell, U., V. J. Fabry, L. Hansson and J.-P. Gattuso (Eds.). (2010). Guide to best practices for ocean acidification research and data reporting, 260 p. Luxembourg: Publications Office of the European Union.
- Sabine, C. L.; Feely, Richard A.; Gruber, Nicolas; Key, Robert M.; Lee, Kitack; Bullister, John L.; et al. (2004). "The Oceanic Sink for Anthropogenic CO
2". Science. 305 (5682): 367–371. Bibcode:2004Sci...305..367S. doi:10.1126/science.1097403. PMID 15256665. - Stone, R. (2007). "A World Without Corals?". Science. 316 (5825): 678–681. doi:10.1126/science.316.5825.678. PMID 17478692.
External links
Scientific sources:
- Ocean Acidification International Coordination Centre (OA-ICC), a project operated by the International Atomic Energy Agency through its Environment Laboratories (Monaco)
- A news stream provided by the Ocean Acidification International Coordination Centre (OA-ICC), provides daily information on ocean acidification (scientific papers, jobs, media coverages, meeting announcements etc.). Subscribe free of charge via email, RSS or Twitter.
- How Acidification Threatens Oceans from the Inside Out Scientific American August 9, 2010 by Marah J. Hardt and Carl Safina
- Ocean acidification due to increasing atmospheric carbon dioxide, report by the Royal Society (UK)
- AR4 WG1 Chapter 5: Oceanic Climate Change and Sea Level, IPCC
- State of the Science FACT SHEET: Ocean acidification, NOAA
- Carbon Dioxide Information Analysis Center (CDIAC), the primary data analysis center of the U.S. Department of Energy (located at Oak Ridge National Laboratory)
- Ocean acidification introduction, USGS
- Climate change threatening the Southern Ocean, report by CSIRO
- The Ocean in a High CO
2 World, an international science symposium series - The Acid Ocean – the Other Problem with CO
2 Emission, David Archer (scientist), a RealClimate discussion - Regularly updated "blog" of ocean acidification publications and news, Jean-Pierre Gattuso
- Task Force on Ocean Acidification in the Pacific, including recent presentations on ocean acidification, Pacific Science Association
- Ocean Acidification, a multimedia, interactive site from The World Ocean Observatory
- Acidic Oceans: Why should we care? Perspectives in ocean science, Andrew Dickson, Scripps Institution of Oceanography
- Climate Change: Coral Reefs on the Edge A video presentation by Prof. Ove Hoegh-Guldberg on impact of ocean acidification on coral reefs
- Life in the Sea Found Its Fate in a Paroxysm of Extinction April 30, 2012
- Ocean acidification virtual lab
- Ocean Acidification: Starting with the Science, a booklet from the Division on Earth & Life Studies of the United States National Research Council (released April 2011)
- Ocean Acidification, a United States National Academy of Sciences/ National Research Council website that includes downloadable figures and interviews with ocean scientists
- Ancient Ocean Acidification Intimates Long Recovery from Climate Change, July 22, 2010
- Acidification alters fish behavior: higher carbon dioxide in oceans may affect brain chemistry February 25, 2012 Science News
- Coordination of international research efforts and synthesis activities in ocean acidification. IMBER/SOLAS
Educational sites:
- Ocean Acidification overview from the Smithsonian Ocean Portal
- Understanding Ocean Acidification -- Educational Site from Channel Islands National Marine Sanctuary -- Education Team
Scientific projects:
- Dr. Francisco Chavez on Ocean Acidification – Smithsonian Ocean Portal
- European Project of Ocean Acidification (EPOCA), a 4-year-long EU initiative to investigate ocean acidification (initiated June 2008)
- Biological Impacts of Ocean Acidification (BIOACID), a German initiative funded by BMBF
- Ocean Acidification Research Programme (UKOARP), a 5-year-long UK initiative funded by NERC, Defra and DECC
- Research Program on Ocean Acidification at the Cluster of Excellence "Future Ocean", Kiel
- Ocean Acidification Research Center at University of Alaska Fairbanks
Government sources:
- Effects of Climate Change and Ocean Acidification on Living Marine Organisms: Hearing before the Subcommittee on Oceans, Atmosphere, Fisheries, and Coast Guard of the Committee on Commerce, Science, and Transportation, United States Senate, One Hundred Tenth Congress, First Session, May 10, 2007
- The Environmental and Economic Impacts of Ocean Acidification: Hearing before the Subcommittee on Oceans, Atmosphere, Fisheries, and Coast Guard of the Committee on Commerce, Science, and Transportation, United States Senate, One Hundred Eleventh Congress, Second Session, April 22, 2010
- Ocean Acidification Congressional Research Service
Popular media sources:
- Threatening Oceans from the Inside Out: How Acidification Affects Marine Life, Scientific American
- "The Darkening Sea, article in The New Yorker magazine, Nov. 20, 2006 (requires registration)
- "Growing Acidity of Oceans May Kill Corals", Washington Post
- "Scientists Grapple with Ocean Acidification", ABC News
- "Ocean Acidification & Climate", by Clayton Sandell ABC News
- A World Without Whales? by Philippe Cousteau, The Huffington Post
- Acid Test: Can we save our oceans from CO
2?, Oceana - The Acid Ocean, Stanford University
- Sea Change (Parts One, Two, and Three), The Seattle Times
Videos on Ocean Acidification:
- The Other CO
2 Problem, an EPOCA-commissioned educational animation created by students from Ridgeway School, Plymouth - Acid Test: The Global Challenge of Ocean Acidification, by Natural Resources Defense Council
- A Sea Change: Imagine a world without fish, an award-winning documentary and related blog about ocean acidification
- Ocean Acidification in a Nutshell, by Greenpeace Aotearoa New Zealand
- Ocean Acidification: An Ecosystem Facing Dissolution by GEOMAR I Helmholtz-Centre for Ocean Research Kiel
- NOVA Lethal Seas, visit a unique coral garden in Papua New Guinea that offers a glimpse of what the seas could be like a half-century from now.
Carbonate system calculators
The following packages calculate the state of the carbonate system in seawater (including pH):
- CO2SYS, a stand-alone executable (also available in a version for Microsoft Excel/VBA)
- seacarb, a R package for Windows, Mac OS X and Linux (also available here)
- CSYS, a Matlab script