Cell signaling
Cell signaling (cell signalling in British English) is part of a complex system of communication that governs basic activities of cells and coordinates cell actions. The ability of cells to perceive and correctly respond to their microenvironment is the basis of development, tissue repair, and immunity as well as normal tissue homeostasis. Errors in cellular information processing are responsible for diseases such as cancer, autoimmunity, and diabetes.[1][2][3] By understanding cell signaling, diseases may be treated more effectively and, theoretically, artificial tissues may be created.[4]
Traditional work in biology has focused on studying individual parts of cell signaling pathways. Systems biology research helps us to understand the underlying structure of cell signaling networks and how changes in these networks may affect the transmission and flow of information (signal transduction). Such networks are complex systems in their organization and may exhibit a number of emergent properties including bistability and ultrasensitivity. Analysis of cell signaling networks requires a combination of experimental and theoretical approaches including the development and analysis of simulations and modeling.[5] Long-range allostery is often a significant component of cell signaling events.[6]
Signaling between cells of one organism and multiple organisms
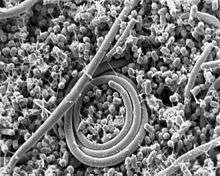
Cell signaling has been most extensively studied in the context of human diseases and signaling between cells of a single organism. However, cell signaling may also occur between the cells of two different organisms. In many mammals, early embryo cells exchange signals with cells of the uterus.[7] In the human gastrointestinal tract, bacteria exchange signals with each other and with human epithelial and immune system cells.[8] For the yeast Saccharomyces cerevisiae during mating, some cells send a peptide signal (mating factor pheromones) into their environment. The mating factor peptide may bind to a cell surface receptor on other yeast cells and induce them to prepare for mating.[9]
Classification
Cell signaling can be classified to be mechanical and biochemical based on the type of the signal. Mechanical signals are the forces exerted on the cell and the forces produced by the cell. These forces can both be sensed and responded by the cells.[10] Biochemical signals are the biochemical molecules such as proteins, lipids, ions and gases. These signals can be categorized based on the distance between signaling and responder cells. Signaling within, between, and among cells is subdivided into the following classifications:
- Intracrine signals are produced by the target cell that stay within the target cell.
- Autocrine signals are produced by the target cell, are secreted, and affect the target cell itself via receptors. Sometimes autocrine cells can target cells close by if they are the same type of cell as the emitting cell. An example of this are immune cells.
- Juxtacrine signals target adjacent (touching) cells. These signals are transmitted along cell membranes via protein or lipid components integral to the membrane and are capable of affecting either the emitting cell or cells immediately adjacent.
- Paracrine signals target cells in the vicinity of the emitting cell. Neurotransmitters represent an example.
- Endocrine signals target distant cells. Endocrine cells produce hormones that travel through the blood to reach all parts of the body.
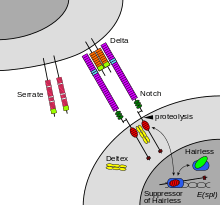
Cells communicate with each other via direct contact (juxtacrine signaling), over short distances (paracrine signaling), or over large distances and/or scales (endocrine signaling).
Some cell–cell communication requires direct cell–cell contact. Some cells can form gap junctions that connect their cytoplasm to the cytoplasm of adjacent cells. In cardiac muscle, gap junctions between adjacent cells allows for action potential propagation from the cardiac pacemaker region of the heart to spread and coordinately cause contraction of the heart.
The notch signaling mechanism is an example of juxtacrine signaling (also known as contact-dependent signaling) in which two adjacent cells must make physical contact in order to communicate. This requirement for direct contact allows for very precise control of cell differentiation during embryonic development. In the worm Caenorhabditis elegans, two cells of the developing gonad each have an equal chance of terminally differentiating or becoming a uterine precursor cell that continues to divide. The choice of which cell continues to divide is controlled by competition of cell surface signals. One cell will happen to produce more of a cell surface protein that activates the Notch receptor on the adjacent cell. This activates a feedback loop or system that reduces Notch expression in the cell that will differentiate and that increases Notch on the surface of the cell that continues as a stem cell.[11]
Many cell signals are carried by molecules that are released by one cell and move to make contact with another cell. Endocrine signals are called hormones. Hormones are produced by endocrine cells and they travel through the blood to reach all parts of the body. Specificity of signaling can be controlled if only some cells can respond to a particular hormone. Paracrine signals such as retinoic acid target only cells in the vicinity of the emitting cell.[12] Neurotransmitters represent another example of a paracrine signal. Some signaling molecules can function as both a hormone and a neurotransmitter. For example, epinephrine and norepinephrine can function as hormones when released from the adrenal gland and are transported to the heart by way of the blood stream. Norepinephrine can also be produced by neurons to function as a neurotransmitter within the brain.[13] Estrogen can be released by the ovary and function as a hormone or act locally via paracrine or autocrine signaling.[14] Active species of oxygen and nitric oxide can also act as cellular messengers. This process is dubbed redox signaling.
Cell signaling in multicellular organisms
In a multicellular organism, signaling between cells occurs either through release into the extracellular space, divided in paracrine signaling (over short distances) and endocrine signaling (over long distances), or by direct contact, known as juxtacrine signaling.[15] Autocrine signaling is a special case of paracrine signaling where the secreting cell has the ability to respond to the secreted signaling molecule.[16] Synaptic signaling is a special case of paracrine signaling (for chemical synapses) or juxtacrine signaling (for electrical synapses) between neurons and target cells. Signaling molecules interact with a target cell as a ligand to cell surface receptors, and/or by entering into the cell through its membrane or endocytosis for intracrine signaling. This generally results in the activation of second messengers, leading to various physiological effects.
A particular molecule is generally used in diverse modes of signaling, and therefore a classification by mode of signaling is not possible. At least three important classes of signaling molecules are widely recognized, although non-exhaustive and with imprecise boundaries, as such membership is non-exclusive and depends on the context:
- Hormones are the major signaling molecules of the endocrine system, though they often regulate each other's secretion via local signaling (e.g. islet of Langerhans cells), and most are also expressed in tissues for local purposes (e.g. angiotensin) or failing that, structurally related molecules are (e.g. PTHrP).
- Neurotransmitters are signaling molecules of the nervous system, also including neuropeptides and neuromodulators. Neurotransmitters like the catecholamines are also secreted by the endocrine system into the systemic circulation.
- Cytokines are signaling molecules of the immune system, with a primary paracrine or juxtacrine role, though they can during significant immune responses have a strong presence in the circulation, with systemic effect (altering iron metabolism or body temperature). Growth factors can be considered as cytokines or a different class.
Signaling molecules can belong to several chemical classes: lipids, phospholipids, amino acids, monoamines, proteins, glycoproteins, or gases. Signaling molecules binding surface receptors are generally large and hydrophilic (e.g. TRH, Vasopressin, Acetylcholine), while those entering the cell are generally small and hydrophobic (e.g. glucocorticoids, thyroid hormones, cholecalciferol, retinoic acid), but important exceptions to both are numerous, and a same molecule can act both via surface receptor or in an intracrine manner to different effects.[16] In intracrine signaling, once inside the cell, a signaling molecule can bind to intracellular receptors, other elements, or stimulate enzyme activity (e.g. gasses). The intracrine action of peptide hormones remains a subject of debate.[17]
Hydrogen sulfide is produced in small amounts by some cells of the human body and has a number of biological signaling functions. Only two other such gases are currently known to act as signaling molecules in the human body: nitric oxide and carbon monoxide.[18]
Receptors for cell motility and differentiation
Cells receive information from their neighbors through a class of proteins known as receptors. Notch is a cell surface protein that functions as a receptor. Animals have a small set of genes that code for signaling proteins that interact specifically with Notch receptors and stimulate a response in cells that express Notch on their surface. Molecules that activate (or, in some cases, inhibit) receptors can be classified as hormones, neurotransmitters, cytokines, and growth factors, in general called receptor ligands. Ligand receptor interactions such as that of the Notch receptor interaction, are known to be main interactions responsible for cell signaling mechanisms and communication.[19]
As shown in Figure 2 (above; left), notch acts as a receptor for ligands that are expressed on adjacent cells. While some receptors are cell surface proteins, others are found inside cells. For example, estrogen is a hydrophobic molecule that can pass through the lipid bilayer of the membranes. As part of the endocrine system, intracellular estrogen receptors from a variety of cell types can be activated by estrogen produced in the ovaries.
A number of transmembrane receptors[20][21] for small molecules and peptide hormones[22] as well as intracellular receptors for steroid hormones exist, giving cells the ability to respond to a great number of hormonal and pharmacological stimuli. In diseases, often, proteins that interact with receptors are aberrantly activated, resulting in constitutively activated downstream signals.[23]
For several types of intercellular signaling molecules that are unable to permeate the hydrophobic cell membrane due to their hydrophilic nature, the target receptor is expressed on the membrane. When such signaling molecule activates its receptor, the signal is carried into the cell usually by means of a second messenger such as cAMP.[24][25]
Signaling pathways
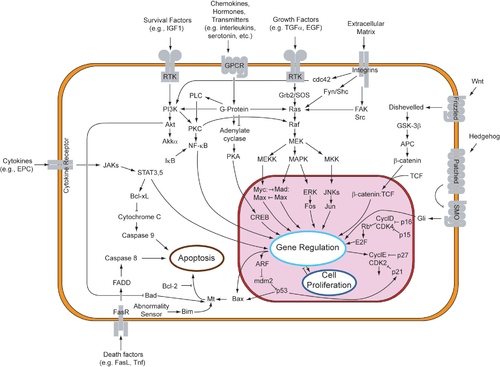

In some cases, receptor activation caused by ligand binding to a receptor is directly coupled to the cell's response to the ligand. For example, the neurotransmitter GABA can activate a cell surface receptor that is part of an ion channel. GABA binding to a GABAA receptor on a neuron opens a chloride-selective ion channel that is part of the receptor. GABAA receptor activation allows negatively charged chloride ions to move into the neuron, which inhibits the ability of the neuron to produce action potentials. However, for many cell surface receptors, ligand-receptor interactions are not directly linked to the cell's response. The activated receptor must first interact with other proteins inside the cell before the ultimate physiological effect of the ligand on the cell's behavior is produced. Often, the behavior of a chain of several interacting cell proteins is altered following receptor activation. The entire set of cell changes induced by receptor activation is called a signal transduction mechanism or pathway.[26]
In the case of Notch-mediated signaling, the signal transduction mechanism can be relatively simple. As shown in Figure 2, activation of Notch can cause the Notch protein to be altered by a protease. Part of the Notch protein is released from the cell surface membrane and takes part in gene regulation. Cell signaling research involves studying the spatial and temporal dynamics of both receptors and the components of signaling pathways that are activated by receptors in various cell types.
A more complex signal transduction pathway is shown in Figure 3. This pathway involves changes of protein–protein interactions inside the cell, induced by an external signal. Many growth factors bind to receptors at the cell surface and stimulate cells to progress through the cell cycle and divide. Several of these receptors are kinases that start to phosphorylate themselves and other proteins when binding to a ligand. This phosphorylation can generate a binding site for a different protein and thus induce protein–protein interaction. In Figure 3, the ligand (called epidermal growth factor (EGF)) binds to the receptor (called EGFR). This activates the receptor to phosphorylate itself. The phosphorylated receptor binds to an adaptor protein (GRB2), which couples the signal to further downstream signaling processes. For example, one of the signal transduction pathways that are activated is called the mitogen-activated protein kinase (MAPK) pathway. The signal transduction component labeled as "MAPK" in the pathway was originally called "ERK," so the pathway is called the MAPK/ERK pathway. The MAPK protein is an enzyme, a protein kinase that can attach phosphate to target proteins such as the transcription factor MYC and, thus, alter gene transcription and, ultimately, cell cycle progression. Many cellular proteins are activated downstream of the growth factor receptors (such as EGFR) that initiate this signal transduction pathway.
Some signaling transduction pathways respond differently, depending on the amount of signaling received by the cell. For instance, the hedgehog protein activates different genes, depending on the amount of hedgehog protein present.
Complex multi-component signal transduction pathways provide opportunities for feedback, signal amplification, and interactions inside one cell between multiple signals and signaling pathways.
Intraspecies and interspecies signaling
Molecular signaling can occur between different organisms, whether unicellular or multicellular, the emitting organism produces the signaling molecule, secrete it into the environment, where it diffuses, and it is sensed or internalized by the receiving organism. In some cases of interspecies signaling, the emitting organism can actually be a host of the receiving organism, or vice versa.
Intraspecies signaling occurs especially in bacteria, yeast, social insects, but also many vertebrates. The signaling molecules used by multicellular organisms are often called pheromones, they can have such purposes as alerting against danger, indicating food supply, or assisting in reproduction.[27] In unicellular organisms such as bacteria, signaling can be used to 'activate' peers from a dormant state, enhance virulence, defend against bacteriophages, etc.[28] In quorum sensing, which is also found in social insects, the multiplicity of individual signals has the potentiality to create a positive feedback loop, generating coordinated response, in this context the signaling molecules are called autoinducers.[29][30][31] This signaling mechanism may have been involved in evolution from unicellular to multicellular organisms.[29][32] Bacteria also use contact-dependent signaling, notably to limit their growth.[33]
Molecular signaling can also occur between individuals of different species, this has been particularly studied in bacteria.[34][35][36] Different bacterial species can coordinate to colonize a host and participate in common quorum sensing.[37] Therapeutic strategies to disrupt this phenomenon are being investigated.[38][39] Interactions mediated through signaling molecules are also thought to occur between the gut flora and their host, as part of their commensal or symbiotic relationship.[39][40] Gram negative microbes deploy bacterial outer membrane vesicles for intra- and inter-species signaling in natural environments and at the host-pathogen interface.
Additionally, interspecies signaling occurs between multicellular organisms. In Vespa mandarinia, individuals release a scent that directs the colony to a food source.[41]
See also
- Biosemiotics
- Molecular cellular cognition
- Cellular communication (biology)
- Crosstalk (biology)
- Bacterial outer membrane vesicles
- Membrane vesicle trafficking
- Host-pathogen interface
- MAPK signaling pathway
- Wnt signaling pathway
- Hedgehog signaling pathway
- Retinoic acid
- TGF beta signaling pathway
- JAK-STAT signaling pathway
- cAMP-dependent pathway
- Protein dynamics
- Signal transduction
- Systems biology
- Lipid signaling
- Redox signaling
- Cell Signaling Technology, an antibody development and production company
- ESIGNET Project, a project to investigate CSNs in silico, funded by the EU under FP6.
- Netpath – A curated resource of signal transduction pathways in humans
- Nanoscale networking – leveraging biological signaling to construct ad hoc in vivo communication networks
- International q-bio Conference on Cellular Information Processing
- Literature-curated human signaling network, the largest human signaling network database
- Soliton model in neuroscience—Physical communication via sound waves in membranes
References
- ↑ "Dynamic aberrant NF-κB spurs tumorigenesis: A new model encompassing the microenvironment". Cytokine Growth Factor Rev. 26: 389–403. Aug 2015. doi:10.1016/j.cytogfr.2015.06.001. PMID 26119834.
- ↑ Wang, K; Grivennikov, SI; Karin, M (2013). "Implications of anti-cytokine therapy in colorectal cancer and autoimmune diseases". Ann. Rheum. Dis. 72 Suppl 2: ii100–3. doi:10.1136/annrheumdis-2012-202201. PMID 23253923.
- ↑ Solinas G, Vilcu C, Neels JG, et al. (2007). "JNK1 in hematopoietically derived cells contributes to diet-induced inflammation and insulin resistance without affecting obesity". Cell Metab. 6 (5): 386–97. doi:10.1016/j.cmet.2007.09.011. PMID 17983584.
- ↑ Smith, RJ; Koobatian, MT; Shahini, A; Swartz, DD; Andreadis, ST (2015). "Capture of endothelial cells under flow using immobilized vascular endothelial growth factor". Biomaterials. 51: 303–12. doi:10.1016/j.biomaterials.2015.02.025. PMC 4361797
. PMID 25771020.
- ↑ Eungdamrong, Narat J.; Iyengar, Ravi (2004-06-01). "Modeling Cell Signaling Networks". Biology of the Cell. 96 (5): 355–362. doi:10.1016/j.biolcel.2004.03.004. ISSN 0248-4900. PMC 3620715
. PMID 15207904.
- ↑ Bu Z, Callaway DJ (2011). "Proteins MOVE! Protein dynamics and long-range allostery in cell signaling". Advances in Protein Chemistry and Structural Biology. Advances in Protein Chemistry and Structural Biology. 83: 163–221. doi:10.1016/B978-0-12-381262-9.00005-7. ISBN 978-0-12-381262-9. PMID 21570668.
- ↑ Mohamed OA, Jonnaert M, Labelle-Dumais C, Kuroda K, Clarke HJ, Dufort D (June 2005). "Uterine Wnt/β-catenin signaling is required for implantation". Proc Natl Acad Sci USA. 102 (24): 8579–84. doi:10.1073/pnas.0500612102. PMC 1150820
. PMID 15930138.
- ↑ Clarke MB, Sperandio V (June 2005). "Events at the host-microbial interface of the gastrointestinal tract III. Cell-to-cell signaling among microbial flora, host, and pathogens: there is a whole lot of talking going on". Am. J. Physiol. Gastrointest. Liver Physiol. 288 (6): G1105–9. doi:10.1152/ajpgi.00572.2004. PMID 15890712.
- ↑ Lin JC, Duell K, Konopka JB (March 2004). "A Microdomain Formed by the Extracellular Ends of the Transmembrane Domains Promotes Activation of the G Protein-Coupled α-Factor Receptor". Mol Cell Biol. 24 (5): 2041–51. doi:10.1128/MCB.24.5.2041-2051.2004. PMC 350546
. PMID 14966283.
- ↑ Miller, Callie Johnson; Davidson, Lance A. (2013). "The interplay between cell signalling and mechanics in developmental processes". Nature Reviews Genetics. 14 (10): 733–744. doi:10.1038/nrg3513. ISSN 1471-0056. PMC 4056017
. PMID 24045690.
- ↑ Greenwald I (June 1998). "LIN-12/Notch signaling: lessons from worms and flies". Genes Dev. 12 (12): 1751–62. doi:10.1101/gad.12.12.1751. PMID 9637676.
- ↑ Duester G (September 2008). "Retinoic Acid Synthesis and Signaling during Early Organogenesis". Cell. 134 (6): 921–31. doi:10.1016/j.cell.2008.09.002. PMC 2632951
. PMID 18805086.
- ↑ Cartford MC, Samec A, Fister M, Bickford PC (2004). "Cerebellar norepinephrine modulates learning of delay classical eyeblink conditioning: Evidence for post-synaptic signaling via PKA". Learn Mem. 11 (6): 732–7. doi:10.1101/lm.83104. PMC 534701
. PMID 15537737.
- ↑ Jesmin S, Mowa CN, Sakuma I, et al. (October 2004). "Aromatase is abundantly expressed by neonatal rat penis but downregulated in adulthood". J Mol Endocrinol. 33 (2): 343–59. doi:10.1677/jme.1.01548. PMID 15525594.
- ↑ Gilbert, Scott F. (2000). "Juxtacrine Signaling". In NCBI bookshelf. Developmental biology (6. ed.). Sunderland, Mass.: Sinauer Assoc. ISBN 0878932437.
- 1 2 Bruce Alberts; et al. (2002). "General Principles of Cell Communication". In NCBI bookshelf. Molecular biology of the cell (4th ed.). New York: Garland Science. ISBN 0815332181.
- ↑ Re, R (October 1999). "The nature of intracrine peptide hormone action". Hypertension. 34 (4 Pt 1): 534–8. doi:10.1161/01.HYP.34.4.534. PMID 10523322.
- ↑ Hausman, Geoffrey M. Cooper, Robert E. (2000). "Signaling Molecules and Their Receptors". In NCBI bookshelf. The cell : a molecular approach (2nd ed.). Washington, D.C.: ASM Press. ISBN 087893300X.
- ↑ Cooper, GM (2000). [Cooper GM. The Cell: A Molecular Approach. 2nd edition. Sunderland (MA): Sinauer Associates; 2000. Functions of Cell Surface Receptors. Available from: https://www.ncbi.nlm.nih.gov/books/NBK9866/ "The Cell: A Molecular Approach"] Check
|url=
value (help). https://www.ncbi.nlm.nih.gov/books/NBK9866/. External link in|website=
(help) - ↑ Domazet I, Holleran BJ, Martin SS, Lavigne P, Leduc R, Escher E, Guillemette G (2009-05-01). "The Second Transmembrane Domain of the Human Type 1 Angiotensin II Receptor Participates in the Formation of the Ligand Binding Pocket and Undergoes Integral Pivoting Movement during the Process of Receptor Activation". J Biol Chem. 284 (18): 11922–9. doi:10.1074/jbc.M808113200. PMC 2673261
. PMID 19276075.
- ↑ Hislop JN, Henry AG, Marchese A, von Zastrow M (2009-07-17). "Ubiquitination Regulates Proteolytic Processing of G Protein-coupled Receptors after Their Sorting to Lysosomes". J Biol Chem. 284 (29): 19361–70. doi:10.1074/jbc.M109.001644. PMC 2740561
. PMID 19433584.
- ↑ Meng H, Zhang X, Hankenson KD, Wang MM (2009-03-20). "Thrombospondin 2 Potentiates Notch3/Jagged1 Signaling". J Biol Chem. 284 (12): 7866–74. doi:10.1074/jbc.M803650200. PMC 2658079
. PMID 19147503.
- ↑ Copland JA, Sheffield-Moore M, Koldzic-Zivanovic N, Gentry S, Lamprou G, Tzortzatou-Stathopoulou F, Zoumpourlis V, Urban RJ, Vlahopoulos SA (June 2009). "Sex steroid receptors in skeletal differentiation and epithelial neoplasia: is tissue-specific intervention possible?". BioEssays. 31 (6): 629–41. doi:10.1002/bies.200800138. PMID 19382224.
- ↑ Goh SL, Looi Y, Shen H, Fang J, Bodner C, Houle M, Ng AC, Screaton RA, Featherstone M (2009-07-10). "Transcriptional Activation by MEIS1A in Response to Protein Kinase A Signaling Requires the Transducers of Regulated CREB Family of CREB Co-activators". J Biol Chem. 284 (28): 18904–12. doi:10.1074/jbc.M109.005090. PMC 2707216
. PMID 19473990.
- ↑ Wojtal KA, Hoekstra D, Van IJzendoorn SC (February 2008). "cAMP-dependent protein kinase A and the dynamics of epithelial cell surface domains: moving membranes to keep in shape". BioEssays. 30 (2): 146–55. doi:10.1002/bies.20705. PMID 18200529.
- ↑ Dinasarapu AR; et al. (2011). "Signaling gateway molecule pages—a data model perspective". Bioinformatics. 27 (12): 1736–1738. doi:10.1093/bioinformatics/btr190. PMC 3106186
. PMID 21505029.
- ↑ Tirindelli, R; Dibattista, M; Pifferi, S; Menini, A (July 2009). "From pheromones to behavior". Physiological reviews. 89 (3): 921–56. doi:10.1152/physrev.00037.2008. PMID 19584317.
- ↑ Mukamolova, GV; Kaprelyants, AS; Young, DI; Young, M; Kell, DB (Jul 21, 1998). "A bacterial cytokine". Proceedings of the National Academy of Sciences of the United States of America. 95 (15): 8916–21. doi:10.1073/pnas.95.15.8916. PMC 21177
. PMID 9671779.
- 1 2 Miller, Melissa B.; Bassler, Bonnie L. (1 October 2001). "Quorum sensing in bacteria". Annual Review of Microbiology. 55 (1): 165–199. doi:10.1146/annurev.micro.55.1.165. PMID 11544353.
- ↑ Kaper, JB; Sperandio, V (June 2005). "Bacterial cell-to-cell signaling in the gastrointestinal tract". Infection and immunity. 73 (6): 3197–209. doi:10.1128/IAI.73.6.3197-3209.2005. PMC 1111840
. PMID 15908344.
- ↑ Camilli, A.; Bonnie L. Bassler (24 February 2006). "Bacterial Small-Molecule Signaling Pathways". Science. 311 (5764): 1113–1116. doi:10.1126/science.1121357. PMC 2776824
. PMID 16497924.
- ↑ Stoka, AM (June 1999). "Phylogeny and evolution of chemical communication: an endocrine approach" (PDF). Journal of molecular endocrinology. 22 (3): 207–25. doi:10.1677/jme.0.0220207. PMID 10343281.
- ↑ Blango, Matthew G; Mulvey, Matthew A (31 March 2009). "Bacterial landlines: contact-dependent signaling in bacterial populations". Current Opinion in Microbiology. 12 (2): 177–181. doi:10.1016/j.mib.2009.01.011. PMC 2668724
. PMID 19246237.
- ↑ Shank, Elizabeth Anne; Kolter, Roberto (31 March 2009). "New developments in microbial interspecies signaling". Current Opinion in Microbiology. 12 (2): 205–214. doi:10.1016/j.mib.2009.01.003. PMC 2709175
. PMID 19251475.
- ↑ Ryan, R. P.; Dow, J. M. (1 July 2008). "Diffusible signals and interspecies communication in bacteria" (PDF). Microbiology. 154 (7): 1845–1858. doi:10.1099/mic.0.2008/017871-0.
- ↑ Ryan, Robert P.; Dow, J. Maxwell (28 February 2011). "Communication with a growing family: diffusible signal factor (DSF) signaling in bacteria". Trends in Microbiology. 19 (3): 145–152. doi:10.1016/j.tim.2010.12.003. PMID 21227698.
- ↑ Déziel, E; Lépine, F; Milot, S; He, J; Mindrinos, MN; Tompkins, RG; Rahme, LG (Feb 3, 2004). "Analysis of Pseudomonas aeruginosa 4-hydroxy-2-alkylquinolines (HAQs) reveals a role for 4-hydroxy-2-heptylquinoline in cell-to-cell communication". Proceedings of the National Academy of Sciences of the United States of America. 101 (5): 1339–44. doi:10.1073/pnas.0307694100. PMC 337054
. PMID 14739337.
- ↑ Federle, Michael J.; Bassler, Bonnie L. (31 October 2003). "Interspecies communication in bacteria". Journal of Clinical Investigation. 112 (9): 1291–1299. doi:10.1172/JCI20195. PMC 228483
. PMID 14597753.
- 1 2 Sperandio, V.; et al. (14 July 2003). "Bacteria-host communication: The language of hormones". Proceedings of the National Academy of Sciences. 100 (15): 8951–8956. doi:10.1073/pnas.1537100100. PMC 166419
. PMID 12847292.
- ↑ Hooper, LV; Gordon, JI (May 11, 2001). "Commensal host-bacterial relationships in the gut". Science. 292 (5519): 1115–1118. doi:10.1126/science.1058709. PMID 11352068.
- ↑ Schueller, Teresa (10 June 2010). "The cues have it; nest-based, cue-mediated recruitment to carbohydrate resources in a swarm-founding social wasp". Naturwissenschaften (Springer-Verlag).
External links
- Signaling Gateway Free summaries of recent research and the Molecule Pages database.
- Protein signaling domains
- NCI-Nature Pathway Interaction Database: authoritative information about signaling pathways in human cells.
- Intercellular Signaling Peptides and Proteins at the US National Library of Medicine Medical Subject Headings (MeSH)
- Cell Communication at the US National Library of Medicine Medical Subject Headings (MeSH)